Abstract
The nature of the first genetic polymer is the subject of major debate1.Although the ‘RNA world’ theory suggests that RNA was the first replicable information carrier of the prebiotic era—that is, prior to the dawn of life2,3—other evidence implies that life may have started with a heterogeneous nucleic acid genetic system that included both RNA and DNA4.Such a theory streamlines the eventual ‘genetic takeover’ of homogeneous DNA from RNA as the principal information-storage molecule, but requires a selective abiotic synthesis of both RNA and DNA building blocks in the same local primordial geochemical scenario. Here we demonstrate a high-yielding, completely stereo-, regio- and furanosyl-selective prebiotic synthesis of the purine deoxyribonucleosides: deoxyadenosine and deoxyinosine. Our synthesis uses key intermediates in the prebiotic synthesis of the canonical pyrimidine ribonucleosides (cytidine and uridine), and we show that, once generated, the pyrimidines persist throughout the synthesis of the purine deoxyribonucleosides, leading to a mixture of deoxyadenosine, deoxyinosine, cytidine and uridine. These results support the notion that purine deoxyribonucleosides and pyrimidine ribonucleosides may have coexisted before the emergence of life5.
This is a preview of subscription content,access via your institution
Access options
Access Nature and 54 other Nature Portfolio journals
Get Nature+, our best-value online-access subscription
24,99 €/ 30 days
cancel any time
Subscribe to this journal
Receive 51 print issues and online access
185,98 € per year
only 3,65 € per issue
Buy this article
- Purchase on SpringerLink
- Instant access to full article PDF
Prices may be subject to local taxes which are calculated during checkout
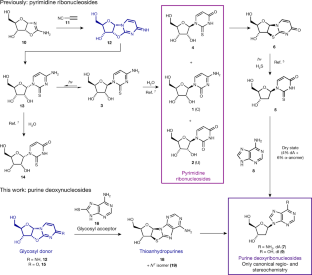
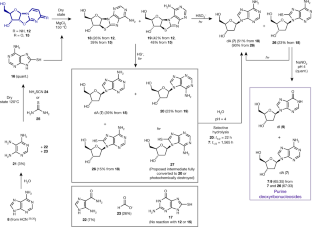
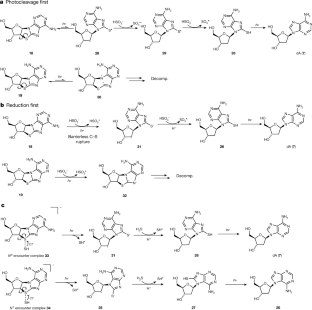
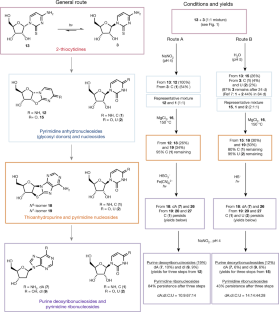
Similar content being viewed by others
Data and materials availability
TheSupplementary Informationavailable for this Article contains all procedures, characterization data, NMR spectra, HPLC traces, X-ray data and Cambridge Crystallographic Data Centre (CCDC) numbers, plus theoretical methods and data. Any additional data are available from the corresponding author upon reasonable request.
Code availability
All custom code used to generate the data in this study is available upon reasonable request.
References
Samanta, B. & Joyce, G. F. A reverse transcriptase ribozyme.eLife6,e31153 (2017).
Gilbert, W. Origin of life: the RNA world.Nature319,618 (1986).
Joyce, G. F. The antiquity of RNA-based evolution.Nature418,214–221 (2002).
Bhowmik, S. & Krishnamurthy, R. The role of sugar-backbone heterogeneity and chimeras in the simultaneous emergence of RNA and DNA.Nat. Chem.11,1009–1018 (2019).
Xu, J., Green, N. J., Gibard, C., Krishnamurthy, R. & Sutherland, J. D. Prebiotic phosphorylation of 2-thiouridine provides either nucleotides or DNA building blocks via photoreduction.Nat. Chem.11,457–462 (2019).
Powner, M. W., Gerland, B. & Sutherland, J. D. Synthesis of activated pyrimidine ribonucleotides in prebiotically plausible conditions.Nature459,239–242 (2009).
Xu, J. et al. A prebiotically plausible synthesis of pyrimidine β-ribonucleosides and their phosphate derivatives involving photoanomerization.Nat. Chem.9,303–309 (2017).
Heuberger, B. D., Pal, A., Del Frate, F., Topkar, V. V. & Szostak, J. W. Replacing uridine with 2-thiouridine enhances the rate and fidelity of nonenzymatic RNA primer extension.J. Am. Chem. Soc.137,2769–2775 (2015).
Walton, T. & Szostak, J. W. A highly reactive imidazolium-bridged dinucleotide intermediate in nonenzymatic RNA primer extension.J. Am. Chem. Soc.138,11996–12002 (2016).
Li, L. et al. Enhanced nonenzymatic RNA copying with 2-aminoimidazole activated nucleotides.J. Am. Chem. Soc.139,1810–1813 (2017).
Fuller, W. D., Orgel, L. E. & Sanchez, R. A. Studies in prebiotic synthesis: VI. Solid-state synthesis of purine nucleosides.J. Mol. Evol.1,249–257 (1972).
Becker, S. et al. A high-yielding, strictly regioselective prebiotic purine nucleoside formation pathway.Science352,833–836 (2016).
Kim, H. & Benner, S. A. Prebiotic stereoselective synthesis of purine and noncanonical pyrimidine nucleotides from nucleobases and phosphorylated carbohydrates.Proc. Natl Acad. Sci. USA114,11315–11320 (2017).
Becker, S. et al. Unified prebiotically plausible synthesis of pyrimidine and purine RNA ribonucleotides.Science366,76–82 (2019).
Teichert, J. S., Kruse, F. M. & Trapp, O. Direct prebiotic pathway to DNA nucleosides.Angew. Chem. Int. Ed.58,9944–9947 (2019).
Reichard, P. From RNA to DNA, why so many ribonucleotide reductases?Science260,1773–1777 (1993).
Leu, K., Obermayer, B., Rajamani, S., Gerland, U. & Chen, I. A. The prebiotic evolutionary advantage of transferring genetic information from RNA to DNA.Nucleic Acids Res.39,8135–8147 (2011).
Sutherland, J. D. & Whitfield, J. N. Prebiotic chemistry: a bioorganic perspective.Tetrahedron53,11493–11527 (1997).
Trevino, S. G., Zhang, N., Elenko, M. P., Lupták, A. & Szostak, J. W. Evolution of functional nucleic acids in the presence of nonheritable backbone heterogeneity.Proc. Natl Acad. Sci. USA108,13492–13497 (2011).
Gavette, J. V., Stoop, M., Hud, N. V. & Krishnamurthy, R. RNA–DNA chimeras in the context of an RNA world transition to an RNA/DNA world.Angew. Chem. Int. Ed.55,13204–13209 (2016).
Schoffstall, A. M. Prebiotic phosphorylation of nucleosides in formamide.Orig. Life7,399–412 (1976).
Lohrmann, R. & Orgel, L. E. Urea-inorganic phosphate mixtures as prebiotic phosphorylating agents.Science171,490–494 (1971).
Patel, B. H., Percivalle, C., Ritson, D. J., Duffy, C. D. & Sutherland, J. D. Common origins of RNA, protein and lipid precursors in a cyanosulfidic protometabolism.Nat. Chem.7,301–307 (2015).
Ishiwata, A., Lee, Y. J. & Ito, Y. Recent advances in stereoselective glycosylation through intramolecular aglycon delivery.Org. Biomol. Chem.8,3596–3608 (2010).
Springsteen, G. & Joyce, G. F. Selective derivatization and sequestration of ribose from a prebiotic mix.J. Am. Chem. Soc.126,9578–9583 (2004).
Anastasi, C., Crowe, M. A., Powner, M. W. & Sutherland, J. D. Direct assembly of nucleoside precursors from two- and three-carbon units.Angew. Chem. Int. Ed.45,6176–6179 (2006).
Vorbrüggen, H. & Ruh-Pohlenz, C.Handbook of Nucleoside Synthesis(Wiley, 2001).
Holm, N. G., Oze, C., Mousis, O., Waite, J. H. & Guilbert-Lepoutre, A. Serpentinization and the formation of H2and CH4on celestial bodies (planets, moons, comets).Astrobiology15,587–600 (2015).
Sanchez, R. A., Ferris, J. P. & Orgel, L. E. Studies in prebiotic synthesis. II: Synthesis of purine precursors and amino acids from aqueous hydrogen cyanide.J. Mol. Biol.80,223–253 (1967).
Hudson, J. S. et al. A unified mechanism for abiotic adenine and purine synthesis in formamide.Angew. Chem. Int. Ed.51,5134–5137 (2012).
Giner-Sorolla, A., Thom, E. & Bendich, A. Studies on the thiation of purines.J. Org. Chem.29,3209–3212 (1964).
Levy, M. & Miller, S. L. The stability of the RNA bases: implications for the origin of life.Proc. Natl Acad. Sci. USA95,7933–7938 (1998).
Ritson, D. J. & Sutherland, J. D. Synthesis of aldehydic ribonucleotide and amino acid precursors by photoredox chemistry.Angew. Chem. Int. Ed.52,5845–5847 (2013).
Robertson, M. P., Levy, M. & Miller, S. L. Prebiotic synthesis of diaminopyrimidine and thiocytosine.J. Mol. Evol.43,543–550 (1996).
Roberts, S. J. et al. Selective prebiotic conversion of pyrimidine and purine anhydronucleosides into Watson–Crick base-pairing arabino-furanosyl nucleosides in water.Nat. Commun.9,4073–4082 (2018).
Ranjan, S., Todd, Z. R., Rimmer, P. B., Sasselov, D. D. & Babbin, A. R. Nitrogen oxide concentrations in natural waters on early Earth.Geochem. Geophys. Geosyst.20,2021–2039 (2019).
Xu, J. et al. Photochemical reductive homologation of hydrogen cyanide using sulfite and ferrocyanide.Chem. Commun.54,5566–5569 (2018).
Marion, G. M., Kargel, J. S., Crowley, J. K. & Catling, D. C. Sulfite–sulfide–sulfate–carbonate equilibria with applications to Mars.Icarus225,342–351 (2013).
Rios, A. C. & Tor, Y. On the origin of the canonical nucleobases: an assessment of selection pressures across chemical and early biological evolution.Isr. J. Chem.53,469–483 (2013).
Rios, A. C., Yu, H. T. & Tor, Y. Hydrolytic fitness ofN-glycosyl bonds: comparing the deglycosylation kinetics of modified, alternative, and native nucleosides.J. Phys. Org. Chem.28,173–180 (2014).
Panzica, R. P., Rousseau, R. J., Robins, R. K. & Townsend, L. B. Relative stability and a quantitative approach to the reaction mechanism of the acid-catalyzed hydrolysis of certain 7-and 9-β-d-ribofuranosylpurines.J. Am. Chem. Soc.94,4708–4714 (1972).
Lindahl, T. & Nyberg, B. Rate of depurination of native deoxyribonucleic acid.Biochemistry11,3610–3618 (1972).
Hättig, C. Structure optimizations for excited states with correlated second-order methods: CC2 and ADC(2).Adv. Quantum Chem.50,37–60 (2005).
Dreuw, A. & Wormit, M. The algebraic diagrammatic construction scheme for the polarization propagator for the calculation of excited states.Wiley Interdiscip. Rev. Comput. Mol. Sci.5,82–95 (2015).
Sauer, M. C., Crowell, R. A. & Shkrob, I. A. Electron photodetachment from aqueous anions. 1. Quantum yields for generation of hydrated electron by 193 and 248 nm laser photoexcitation of miscellaneous inorganic anions.J. Phys. Chem. A108,5490–5502 (2004).
Pascoe, D. J., Ling, K. B. & Cockroft, S. L. The origin of chalcogen-bonding interactions.J. Am. Chem. Soc.139,15160–15167 (2017).
Kim, S. C., O’Flaherty, D. K., Zhou, L., Lelyveld, V. S. & Szostak, J. W. Inosine, but none of the 8-oxo-purines, is a plausible component of a primordial version of RNA.Proc. Natl Acad. Sci. USA115,13318–13323 (2018).
Karran, P. & Lindahl, T. Hypoxanthine in deoxyribonucleic acid: generation by heat-induced hydrolysis of adenine residues and release in free form by a deoxyribonucleic acid glycosylase from calf thymus.Biochemistry19,6005–6011 (1980).
Shapiro, R. & Pohl, S. H. Reaction of ribonucleosides with nitrous acid. Side products and kinetics.Biochemistry7,448–455 (1968).
Mariani, A. D., Russell, A., Javelle, T. & Sutherland, J. D. A light-releasable potentially prebiotic nucleotide activating agent.J. Am. Chem. Soc.140,8657–8661 (2018).
Acknowledgements
The authors thank all JDS group members for discussions. This research was supported by the Medical Research Council (MC_UP_A024_1009), the Simons Foundation (290362 to J.D.S., 494188 to R.S.), and a grant from the National Science Centre Poland (2016/23/B/ST4/01048 to R.W.G.). M.J.J. acknowledges the support of the ‘Diamond Grant’ (0144/DIA/2017/46) from the Polish Ministry of Science and Higher Education and a computational grant from Wrocław Centre of Networking and Supercomputing (WCSS). R.S. thanks the Foundation for Polish Science for support from the START Fellowship.
Author information
Authors and Affiliations
Contributions
Experimental contributions by J.X., V.C., N.J.G., D.A.R. and A.D.B. Theoretical contributions by M.J.J., R.W.G. and R.S. Crystallography by A.D.B. This work was supervised by J.D.S. All authors co-wrote the manuscript.
Corresponding author
Ethics declarations
Competing interests
The authors declare no competing interests.
Additional information
Peer review informationNaturethanks Hannes Mutschler and Yitzhak Tor for their contribution to the peer review of this work.
Publisher’s noteSpringer Nature remains neutral with regard to jurisdictional claims in published maps and institutional affiliations.
Extended data figures and tables
Extended Data Fig. 1 A summary of the main findings of the work.
Previously, a prebiotically plausible synthesis of β-ribopyrimdines C and U has been identified using α-thiocytidine. Herein, we demonstrate that the same intermediate can undergo a distinct prebiotically plausible process that could have happened in a similar—or the same—environment. This process furnishes β-D-N9-deoxyribopurine nucleosides dA and dI alongside the pyrimidines. Remarkable selectivity enforced by UV irradiation and hydrolysis operates throughout the reported ribosylpyrimidine synthesis and the discovered deoxyribosylpurine synthesis, resulting in a set of nucleosides with only the canonical regio- and stereochemistry. The coexistence in one location of a set of nucleosides similar to this is thought to be a precondition for the spontaneous emergence of life on Earth6,47.
Extended Data Fig. 21H NMR spectra of conversion of α-anhydrouridine (15) from α-thiouridine (14).
a,1H NMR spectrum of15.b,1H NMR spectrum of the reaction mixture after heating14in H2O.c,1H NMR spectrum of the reaction mixture after heating14in formamide. f1, chemical shift (δ).
Extended Data Fig. 31H NMR spectra of photoreduction ofN7-8,2′-anhydro-thioadenosine (18) andN9-8,2′-anhydro-thioadenosine (19) mixture with bisulfite.
a,1H NMR spectrum of the crude mixture before irradiation; the ratio ofN7:N9isomer was 4:5.b,1H NMR spectrum of the mixture after irradiation for 7 h; theN9isomers dA (7) and26are the only detectable products. f1, chemical shift (δ).
Extended Data Fig. 4 Potential energy surfaces and S1/S0state crossings of the key photochemical steps in deoxyadenosine synthesis calculated using ADC(2) and the ma-def2-TZVP basis set.
SeeSupplementary Informationfor details.a,Potential energy profile of UV-induced C–S bond scission of18.C–S bond opening may spontaneously occur in18,leading to a peaked S1/S0state crossing; however, a reducing agent is necessary to maintain that geometry after reaching the S0state.b,Potential energy profile of UV-induced N7–C8 bond scission of19.N7–C8 bond rupture is the lowest-energy photochemical process in19and results in destruction of the purine ring.c,d,Potential energy profiles of the UV-induced C–S bond scission of encounter complexes18(c) and19(d) with HS−.Photochemical C–S bond rupture induced by charge transfer from HS−to a chromophore and is a barrierless process.
Extended Data Fig. 5 Equilibrium geometries of C2, S8 radical anion (31) and C8, N9 radical anion (32).
Radical anions may be formed after accepting a hydrated electron from the environment. The adiabatic electron affinities are calculated using ωB97X-D/IEFPCM and the ma-def2-TZVP basis set.
Extended Data Fig. 61H NMR spectra for the reactions of deoxyadenosine (dA, 7) and cytidine (C, 1) with nitrous acid.
a,1H NMR spectrum of the mixture of dA (7) and C (1).b,1H NMR spectrum of the reaction mixture after 4 d, showing that the ratio of the four (deoxy)nucleosides dA (7), deoxyinosine (dI,9), C (1), and uridine (U,2) is 30:17:42:11. f1, chemical shift (δ).
Extended Data Fig. 71H NMR spectra for stability study of cytidine (C; 1) and uridine (U; 2) at 254 nm irradiation with bisulfite.
a,1H NMR spectrum of the mixture of C (1), bisulfite and K4Fe(CN)6in the dark.b,As ina,after 10 h of irradiation.c,1H NMR spectrum of the mixture of U (2), bisulfite and K4Fe(CN)6in the dark.d,As inc,after 10 h of irradiation.e,1H NMR spectrum of the mixture of C (1), U (2),N9-thioanhydroadenosine (18), bisulfite and K4Fe(CN)6in the dark.f,As ine,after 10 h of irradiation. f1, chemical shift (δ).
Extended Data Fig. 81H NMR spectra for sequential reactions with the mixture of α-anhydrouridine (15), C (1) and U (2).
a,1H NMR spectrum of the mixture after heating with 8-mercaptoadenine (16) and magnesium chloride at 150 °C for 1.5 d.b,As ina,after irradiation with hydrogen sulfide at 254 nm.c,As ina,after reacting with nitrous acid for 2 d; dA (7):dI (9):C (1):U (2) = 14:14:44:28). f1, chemical shift (δ).
Supplementary information
Supplementary Information
This file contains Supplementary Materials and Methods, Supplementary Figures 1-56, Supplementary Tables 1-9 and Supplementary References.
Rights and permissions
About this article
Cite this article
Xu, J., Chmela, V., Green, N.et al.Selective prebiotic formation of RNA pyrimidine and DNA purine nucleosides. Nature582,60–66 (2020). https://doi.org/10.1038/s41586-020-2330-9
Received:
Accepted:
Published:
Issue Date:
DOI:https://doi.org/10.1038/s41586-020-2330-9
This article is cited by
-
Nucleoside Phosphorylases make N7-xanthosine
Nature Communications(2024)
-
Primitive purine biosynthesis connects ancient geochemistry to modern metabolism
Nature Ecology & Evolution(2024)
-
Cosmic dust fertilization of glacial prebiotic chemistry on early Earth
Nature Astronomy(2024)
-
A green one-pot synthetic protocol of hexahydropyrimido[4,5-d]pyrimidin-4(1H)-one derivatives: molecular docking, ADMET, anticancer and antimicrobial studies
Molecular Diversity(2024)
-
Tides over titanium
Nature Synthesis(2023)
Comments
By submitting a comment you agree to abide by ourTermsandCommunity Guidelines.If you find something abusive or that does not comply with our terms or guidelines please flag it as inappropriate.