Biochemistry
Part of a series on |
Biochemistry |
---|
![]() |
Biochemistryorbiological chemistryis the study ofchemical processeswithin and relating to livingorganisms.[1]A sub-discipline of bothchemistryandbiology,biochemistry may be divided into three fields:structural biology,enzymology,andmetabolism.Over the last decades of the 20th century, biochemistry has become successful at explaining living processes through these three disciplines. Almost allareas of the life sciencesare being uncovered and developed through biochemical methodology and research.[2]Biochemistry focuses on understanding the chemical basis which allowsbiological moleculesto give rise to the processes that occur within livingcellsand between cells,[3]in turn relating greatly to the understanding oftissuesandorgansas well as organism structure and function.[4]Biochemistry is closely related tomolecular biology,the study of themolecularmechanisms of biological phenomena.[5]
Much of biochemistry deals with the structures, functions, and interactions of biologicalmacromoleculessuch asproteins,nucleic acids,carbohydrates,andlipids.They provide the structure of cells and perform many of the functions associated with life.[6]The chemistry of the cell also depends upon the reactions of smallmoleculesandions.These can beinorganic(for example,waterandmetalions) ororganic(for example, theamino acids,which are used tosynthesize proteins).[7]The mechanisms used bycells to harness energyfrom their environment viachemical reactionsare known asmetabolism.The findings of biochemistry are applied primarily inmedicine,nutritionandagriculture.In medicine,biochemistsinvestigate the causes andcuresofdiseases.[8]Nutrition studies how to maintain health and wellness and also the effects ofnutritional deficiencies.[9]In agriculture, biochemists investigatesoilandfertilizerswith the goal of improving crop cultivation, crop storage, andpest control.In recent decades, biochemical principles and methods have been combined with problem-solving approaches fromengineeringto manipulate living systems in order to produce useful tools for research, industrial processes, and diagnosis and control of disease—the discipline ofbiotechnology.
History
[edit]
At its most comprehensive definition, biochemistry can be seen as a study of the components and composition of living things and how they come together to become life. In this sense, the history of biochemistry may therefore go back as far as theancient Greeks.[10]However, biochemistry as a specificscientific disciplinebegan sometime in the 19th century, or a little earlier, depending on which aspect of biochemistry is being focused on. Some argued that the beginning of biochemistry may have been the discovery of the firstenzyme,diastase(now calledamylase), in 1833 byAnselme Payen,[11]while others consideredEduard Buchner's first demonstration of a complex biochemical processalcoholic fermentationin cell-free extracts in 1897 to be the birth of biochemistry.[12][13]Some might also point as its beginning to the influential 1842 work byJustus von Liebig,Animal chemistry, or,Organic chemistryin its applications tophysiologyandpathology,which presented a chemical theory of metabolism,[10]or even earlier to the 18th century studies onfermentationandrespirationbyAntoine Lavoisier.[14][15]Many other pioneers in the field who helped to uncover the layers of complexity of biochemistry have been proclaimed founders of modern biochemistry.Emil Fischer,who studied the chemistry ofproteins,[16]andF. Gowland Hopkins,who studiedenzymesand the dynamic nature of biochemistry, represent two examples of early biochemists.[17]
The term "biochemistry" was first used when Vinzenz Kletzinsky (1826–1882) had his "Compendium der Biochemie" printed in Vienna in 1858; it derived from a combination ofbiologyandchemistry.In 1877,Felix Hoppe-Seylerused the term (biochemiein German) as a synonym forphysiological chemistryin the foreword to the first issue ofZeitschrift für Physiologische Chemie(Journal of Physiological Chemistry) where he argued for the setting up of institutes dedicated to this field of study.[18][19]The GermanchemistCarl Neuberghowever is often cited to have coined the word in 1903,[20][21][22]while some credited it toFranz Hofmeister.[23]
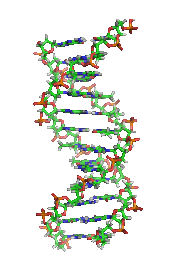
It was once generally believed that life and its materials had some essential property or substance (often referred to as the "vital principle") distinct from any found in non-living matter, and it was thought that only living beings could produce the molecules of life.[25]In 1828,Friedrich Wöhlerpublished a paper on hisserendipitousureasynthesisfrompotassium cyanateandammonium sulfate;some regarded that as a direct overthrow of vitalism and the establishment oforganic chemistry.[26][27]However, the Wöhler synthesis has sparked controversy as some reject the death of vitalism at his hands.[28]Since then, biochemistry has advanced, especially since the mid-20th century, with the development of new techniques such aschromatography,X-ray diffraction,dual polarisation interferometry,NMR spectroscopy,radioisotopic labeling,electron microscopyandmolecular dynamicssimulations. These techniques allowed for the discovery and detailed analysis of many molecules andmetabolic pathwaysof thecell,such asglycolysisand theKrebs cycle(citric acid cycle), and led to an understanding of biochemistry on a molecular level.[citation needed]
Another significant historic event in biochemistry is the discovery of thegene,and its role in the transfer of information in the cell. In the 1950s,James D. Watson,Francis Crick,Rosalind FranklinandMaurice Wilkinswere instrumental in solvingDNA structureand suggesting its relationship with the genetic transfer of information.[29]In 1958,George BeadleandEdward Tatumreceived theNobel Prizefor work in fungi showing thatone gene produces one enzyme.[30]In 1988,Colin Pitchforkwas the first person convicted of murder withDNAevidence, which led to the growth offorensic science.[31]More recently,Andrew Z. FireandCraig C. Melloreceived the2006 Nobel Prizefor discovering the role ofRNA interference(RNAi) in the silencing ofgene expression.[32]
Starting materials: the chemical elements of life
[edit]
Around two dozenchemical elementsare essential to various kinds ofbiological life.Most rare elements on Earth are not needed by life (exceptions beingseleniumandiodine),[33]while a few common ones (aluminumandtitanium) are not used. Most organisms share element needs, but there are a few differences betweenplantsandanimals.For example, ocean algae usebromine,but land plants and animals do not seem to need any. All animals requiresodium,but is not an essential element for plants. Plants needboronandsilicon,but animals may not (or may need ultra-small amounts).[citation needed]
Just six elements—carbon,hydrogen,nitrogen,oxygen,calciumandphosphorus—make up almost 99% of the mass of living cells, including those in the human body (seecomposition of the human bodyfor a complete list). In addition to the six major elements that compose most of the human body, humans require smaller amounts of possibly 18 more.[34]
Biomolecules
[edit]The 4 main classes of molecules in biochemistry (often calledbiomolecules) arecarbohydrates,lipids,proteins,andnucleic acids.[35]Many biological molecules arepolymers:in this terminology,monomersare relatively small macromolecules that are linked together to create largemacromoleculesknown as polymers. When monomers are linked together to synthesize abiological polymer,they undergo a process calleddehydration synthesis.Different macromolecules can assemble in larger complexes, often needed forbiological activity.
Carbohydrates
[edit]Two of the main functions of carbohydrates are energy storage and providing structure. One of the commonsugarsknown asglucoseis a carbohydrate, but not all carbohydrates are sugars. There are more carbohydrates on Earth than any other known type of biomolecule; they are used to store energy andgenetic information,as well as play important roles in cell tocell interactionsandcommunications.[citation needed]
The simplest type of carbohydrate is amonosaccharide,which among other properties containscarbon,hydrogen,andoxygen,mostly in a ratio of 1:2:1 (generalized formula CnH2nOn,wherenis at least 3).Glucose(C6H12O6) is one of the most important carbohydrates; others includefructose(C6H12O6), the sugar commonly associated with thesweet tasteoffruits,[36][a]anddeoxyribose(C5H10O4), a component ofDNA.A monosaccharide can switch betweenacyclic (open-chain) formand acyclicform. The open-chain form can be turned into a ring of carbon atoms bridged by anoxygenatom created from thecarbonyl groupof one end and thehydroxylgroup of another. The cyclic molecule has ahemiacetalorhemiketalgroup, depending on whether the linear form was analdoseor aketose.[38]
In these cyclic forms, the ring usually has5or6atoms. These forms are calledfuranosesandpyranoses,respectively—by analogy withfuranandpyran,the simplest compounds with the same carbon-oxygen ring (although they lack the carbon-carbondouble bondsof these two molecules). For example, the aldohexoseglucosemay form a hemiacetal linkage between the hydroxyl on carbon 1 and the oxygen on carbon 4, yielding a molecule with a 5-membered ring, calledglucofuranose.The same reaction can take place between carbons 1 and 5 to form a molecule with a 6-membered ring, calledglucopyranose.Cyclic forms with a 7-atom ring calledheptosesare rare.[citation needed]
Two monosaccharides can be joined by aglycosidicorester bondinto adisaccharidethrough adehydration reactionduring which a molecule of water is released. The reverse reaction in which the glycosidic bond of a disaccharide is broken into two monosaccharides is termedhydrolysis.The best-known disaccharide issucroseor ordinarysugar,which consists of aglucosemolecule and afructosemolecule joined. Another important disaccharide islactosefound in milk, consisting of a glucose molecule and agalactosemolecule. Lactose may be hydrolysed bylactase,and deficiency in this enzyme results inlactose intolerance.
When a few (around three to six) monosaccharides are joined, it is called anoligosaccharide(oligo-meaning "few" ). These molecules tend to be used as markers andsignals,as well as having some other uses.[39]Many monosaccharides joined form apolysaccharide.They can be joined in one long linear chain, or they may bebranched.Two of the most common polysaccharides arecelluloseandglycogen,both consisting of repeating glucosemonomers.Celluloseis an important structural component of plant'scell wallsandglycogenis used as a form of energy storage in animals.
Sugarcan be characterized by havingreducingor non-reducing ends. Areducing endof a carbohydrate is a carbon atom that can be in equilibrium with the open-chainaldehyde(aldose) or keto form (ketose). If the joining of monomers takes place at such a carbon atom, the freehydroxy groupof thepyranoseorfuranoseform is exchanged with an OH-side-chain of another sugar, yielding a fullacetal.This prevents opening of the chain to the aldehyde or keto form and renders the modified residue non-reducing. Lactose contains a reducing end at its glucose moiety, whereas the galactose moiety forms a full acetal with the C4-OH group of glucose.Saccharosedoes not have a reducing end because of full acetal formation between the aldehyde carbon of glucose (C1) and the keto carbon of fructose (C2).
Lipids
[edit]
Lipidscomprise a diverse range ofmoleculesand to some extent is a catchall for relatively water-insoluble ornonpolarcompounds of biological origin, includingwaxes,fatty acids,fatty-acid derivedphospholipids,sphingolipids,glycolipids,andterpenoids(e.g.,retinoidsandsteroids). Some lipids are linear, open-chainaliphaticmolecules, while others have ring structures. Some arearomatic(with a cyclic [ring] and planar [flat] structure) while others are not. Some are flexible, while others are rigid.[42]
Lipids are usually made from one molecule ofglycerolcombined with other molecules. Intriglycerides,the main group of bulk lipids, there is one molecule of glycerol and threefatty acids.Fatty acids are considered the monomer in that case, and maybesaturated(nodouble bondsin the carbon chain) or unsaturated (one or more double bonds in the carbon chain).[citation needed]
Most lipids have somepolarcharacter and are largely nonpolar. In general, the bulk of their structure is nonpolar orhydrophobic( "water-fearing" ), meaning that it does not interact well with polar solvents likewater.Another part of their structure is polar orhydrophilic( "water-loving" ) and will tend to associate with polar solvents like water. This makes themamphiphilicmolecules (having both hydrophobic and hydrophilic portions). In the case ofcholesterol,the polar group is a mere –OH (hydroxyl or alcohol).[citation needed]
In the case of phospholipids, the polar groups are considerably larger and more polar, as described below.
Lipids are an integral part of our daily diet. Mostoilsandmilk productsthat we use for cooking and eating likebutter,cheese,gheeetc. are composed offats.Vegetable oilsare rich in variouspolyunsaturated fatty acids(PUFA). Lipid-containing foods undergo digestion within the body and are broken into fatty acids and glycerol, the final degradation products of fats and lipids. Lipids, especiallyphospholipids,are also used in variouspharmaceutical products,either as co-solubilizers (e.g. in parenteral infusions) or else asdrug carriercomponents (e.g. in aliposomeortransfersome).
Proteins
[edit]
Proteinsare very large molecules—macro-biopolymers—made from monomers calledamino acids.An amino acid consists of an alpha carbon atom attached to anaminogroup, –NH2,acarboxylic acidgroup, –COOH (although these exist as –NH3+and –COO−under physiologic conditions), a simplehydrogen atom,and a side chain commonly denoted as "–R". The side chain "R" is different for each amino acid of which there are 20standard ones.It is this "R" group that makes each amino acid different, and the properties of the side chains greatly influence the overallthree-dimensional conformationof a protein. Some amino acids have functions by themselves or in a modified form; for instance,glutamatefunctions as an importantneurotransmitter.Amino acids can be joined via apeptide bond.In thisdehydrationsynthesis, awater moleculeis removed and the peptide bond connects thenitrogenof one amino acid's amino group to the carbon of the other's carboxylic acid group. The resulting molecule is called adipeptide,and short stretches of amino acids (usually, fewer than thirty) are calledpeptidesorpolypeptides.Longer stretches merit the titleproteins.As an example, the important bloodserumproteinalbumincontains 585amino acid residues.[43]


Proteins can have structural and/or functional roles. For instance, movements of the proteinsactinandmyosinultimately are responsible for the contraction of skeletal muscle. One property many proteins have is that they specifically bind to a certain molecule or class of molecules—they may beextremelyselective in what they bind.Antibodiesare an example of proteins that attach to one specific type of molecule. Antibodies are composed of heavy and light chains. Two heavy chains would be linked to two light chains throughdisulfide linkagesbetween their amino acids. Antibodies are specific through variation based on differences in the N-terminal domain.[44]
Theenzyme-linked immunosorbent assay(ELISA), which uses antibodies, is one of the most sensitive tests modern medicine uses to detect various biomolecules. Probably the most important proteins, however, are theenzymes.Virtually every reaction in a living cell requires an enzyme to lower theactivation energyof the reaction. These molecules recognize specific reactant molecules calledsubstrates;they thencatalyzethe reaction between them. By lowering theactivation energy,the enzyme speeds up that reaction by a rate of 1011or more; a reaction that would normally take over 3,000 years to complete spontaneously might take less than a second with an enzyme. The enzyme itself is not used up in the process and is free to catalyze the same reaction with a new set of substrates. Using various modifiers, the activity of the enzyme can be regulated, enabling control of the biochemistry of the cell as a whole.
The structure of proteins is traditionally described in a hierarchy of four levels. Theprimary structureof a protein consists of its linear sequence of amino acids; for instance, "alanine-glycine-tryptophan-serine-glutamate-asparagine-glycine-lysine-...".Secondary structureis concerned with local morphology (morphology being the study of structure). Some combinations of amino acids will tend to curl up in a coil called anα-helixor into a sheet called aβ-sheet;some α-helixes can be seen in the hemoglobin schematic above.Tertiary structureis the entire three-dimensional shape of the protein. This shape is determined by the sequence of amino acids. In fact, a single change can change the entire structure. The alpha chain of hemoglobin contains 146 amino acid residues; substitution of theglutamateresidue at position 6 with avalineresidue changes the behavior of hemoglobin so much that it results insickle-cell disease.Finally,quaternary structureis concerned with the structure of a protein with multiple peptide subunits, like hemoglobin with its four subunits. Not all proteins have more than one subunit.[45]


Ingested proteins are usually broken up into single amino acids or dipeptides in thesmall intestineand then absorbed. They can then be joined to form new proteins. Intermediate products of glycolysis, the citric acid cycle, and thepentose phosphate pathwaycan be used to form all twenty amino acids, and most bacteria and plants possess all the necessary enzymes to synthesize them. Humans and other mammals, however, can synthesize only half of them. They cannot synthesizeisoleucine,leucine,lysine,methionine,phenylalanine,threonine,tryptophan,andvaline.Because they must be ingested, these are theessential amino acids.Mammals do possess the enzymes to synthesizealanine,asparagine,aspartate,cysteine,glutamate,glutamine,glycine,proline,serine,andtyrosine,the nonessential amino acids. While they can synthesizearginineandhistidine,they cannot produce it in sufficient amounts for young, growing animals, and so these are often considered essential amino acids.
If the amino group is removed from an amino acid, it leaves behind a carbon skeleton called an α-keto acid.Enzymes calledtransaminasescan easily transfer the amino group from one amino acid (making it an α-keto acid) to another α-keto acid (making it an amino acid). This is important in the biosynthesis of amino acids, as for many of the pathways, intermediates from other biochemical pathways are converted to the α-keto acid skeleton, and then an amino group is added, often viatransamination.The amino acids may then be linked together to form a protein.
A similar process is used to break down proteins. It is first hydrolyzed into its component amino acids. Freeammonia(NH3), existing as theammoniumion (NH4+) in blood, is toxic to life forms. A suitable method for excreting it must therefore exist. Different tactics have evolved in different animals, depending on the animals' needs.Unicellularorganisms release the ammonia into the environment. Likewise,bony fishcan release ammonia into the water where it is quickly diluted. In general, mammals convert ammonia into urea, via theurea cycle.
In order to determine whether two proteins are related, or in other words to decide whether they are homologous or not, scientists use sequence-comparison methods. Methods likesequence alignmentsandstructural alignmentsare powerful tools that help scientists identifyhomologiesbetween related molecules. The relevance of finding homologies among proteins goes beyond forming an evolutionary pattern ofprotein families.By finding how similar two protein sequences are, we acquire knowledge about their structure and therefore their function.
Nucleic acids
[edit]
Nucleic acids,so-called because of their prevalence in cellularnuclei,is the generic name of the family ofbiopolymers.They are complex, high-molecular-weight biochemical macromolecules that can conveygenetic informationin all living cells and viruses.[2]The monomers are callednucleotides,and each consists of three components: a nitrogenous heterocyclicbase(either apurineor apyrimidine), a pentose sugar, and aphosphategroup.[46]

The most common nucleic acids aredeoxyribonucleic acid(DNA) andribonucleic acid(RNA). Thephosphate groupand the sugar of each nucleotide bond with each other to form the backbone of the nucleic acid, while the sequence of nitrogenous bases stores the information. The most common nitrogenous bases areadenine,cytosine,guanine,thymine,anduracil.Thenitrogenous basesof each strand of a nucleic acid will formhydrogen bondswith certain other nitrogenous bases in a complementary strand of nucleic acid. Adenine binds with thymine and uracil, thymine binds only with adenine, and cytosine and guanine can bind only with one another. Adenine, thymine, and uracil contain two hydrogen bonds, while hydrogen bonds formed between cytosine and guanine are three.
Aside from the genetic material of the cell, nucleic acids often play a role assecond messengers,as well as forming the base molecule foradenosine triphosphate(ATP), the primary energy-carrier molecule found in all living organisms. Also, the nitrogenous bases possible in the two nucleic acids are different: adenine, cytosine, and guanine occur in both RNA and DNA, while thymine occurs only in DNA and uracil occurs in RNA.
Metabolism
[edit]Carbohydrates as energy source
[edit]Glucose is an energy source in most life forms. For instance, polysaccharides are broken down into their monomers byenzymes(glycogen phosphorylaseremoves glucose residues from glycogen, a polysaccharide). Disaccharides like lactose or sucrose are cleaved into their two component monosaccharides.[47]
Glycolysis (anaerobic)
[edit]![]() |
Glucose is mainly metabolized by a very important ten-steppathwaycalledglycolysis,the net result of which is to break down one molecule of glucose into two molecules ofpyruvate.This also produces a net two molecules ofATP,the energy currency of cells, along with two reducing equivalents of convertingNAD+(nicotinamide adenine dinucleotide: oxidized form) to NADH (nicotinamide adenine dinucleotide: reduced form). This does not require oxygen; if no oxygen is available (or the cell cannot use oxygen), the NAD is restored by converting the pyruvate tolactate (lactic acid)(e.g. in humans) or toethanolplus carbon dioxide (e.g. inyeast). Other monosaccharides like galactose and fructose can be converted into intermediates of the glycolytic pathway.[48]
Aerobic
[edit]Inaerobiccells with sufficientoxygen,as in most human cells, the pyruvate is further metabolized. It is irreversibly converted toacetyl-CoA,giving off one carbon atom as the waste productcarbon dioxide,generating another reducing equivalent asNADH.The two molecules acetyl-CoA (from one molecule of glucose) then enter thecitric acid cycle,producing two molecules of ATP, six more NADH molecules and two reduced (ubi)quinones (viaFADH2as enzyme-bound cofactor), and releasing the remaining carbon atoms as carbon dioxide. The produced NADH and quinol molecules then feed into the enzyme complexes of the respiratory chain, anelectron transport systemtransferring the electrons ultimately to oxygen and conserving the released energy in the form of a proton gradient over a membrane (inner mitochondrial membranein eukaryotes). Thus, oxygen is reduced to water and the original electron acceptors NAD+andquinoneare regenerated. This is why humans breathe in oxygen and breathe out carbon dioxide. The energy released from transferring the electrons from high-energy states in NADH and quinol is conserved first as proton gradient and converted to ATP via ATP synthase. This generates an additional28molecules of ATP (24 from the 8 NADH + 4 from the 2 quinols), totaling to 32 molecules of ATP conserved per degraded glucose (two from glycolysis + two from the citrate cycle).[49]It is clear that using oxygen to completely oxidize glucose provides an organism with far more energy than any oxygen-independent metabolic feature, and this is thought to be the reason why complex life appeared only after Earth's atmosphere accumulated large amounts of oxygen.
Gluconeogenesis
[edit]Invertebrates,vigorously contractingskeletal muscles(during weightlifting or sprinting, for example) do not receive enough oxygen to meet the energy demand, and so they shift toanaerobic metabolism,converting glucose to lactate. The combination of glucose from noncarbohydrates origin, such as fat and proteins. This only happens whenglycogensupplies in the liver are worn out. The pathway is a crucial reversal ofglycolysisfrom pyruvate to glucose and can use many sources like amino acids, glycerol andKrebs Cycle.Large scale protein and fatcatabolismusually occur when those suffer from starvation or certain endocrine disorders.[50]Theliverregenerates the glucose, using a process calledgluconeogenesis.This process is not quite the opposite of glycolysis, and actually requires three times the amount of energy gained from glycolysis (six molecules of ATP are used, compared to the two gained in glycolysis). Analogous to the above reactions, the glucose produced can then undergo glycolysis in tissues that need energy, be stored as glycogen (orstarchin plants), or be converted to other monosaccharides or joined into di- or oligosaccharides. The combined pathways of glycolysis during exercise, lactate's crossing via the bloodstream to the liver, subsequent gluconeogenesis and release of glucose into the bloodstream is called theCori cycle.[51]
Relationship to other "molecular-scale" biological sciences
[edit]![]() | This section has multiple issues.Please helpimprove itor discuss these issues on thetalk page.(Learn how and when to remove these messages)
|

Researchers in biochemistry use specific techniques native to biochemistry, but increasingly combine these with techniques and ideas developed in the fields ofgenetics,molecular biology,andbiophysics.There is not a defined line between these disciplines. Biochemistry studies thechemistryrequired for biological activity of molecules, molecular biology studies their biological activity,geneticsstudies their heredity, which happens to be carried by theirgenome.This is shown in the following schematic that depicts one possible view of the relationships between the fields:
- Biochemistryis the study of the chemical substances and vital processes occurring in liveorganisms.Biochemistsfocus heavily on the role, function, and structure ofbiomolecules.The study of the chemistry behind biological processes and the synthesis of biologically active molecules are applications of biochemistry. Biochemistry studies life at the atomic and molecular level.
- Geneticsis the study of the effect of genetic differences in organisms. This can often be inferred by the absence of a normal component (e.g. onegene). The study of "mutants"– organisms that lack one or more functional components with respect to the so-called"wild type"or normalphenotype.Genetic interactions (epistasis) can often confound simple interpretations of such "knockout"studies.
- Molecular biologyis the study of molecular underpinnings of the biological phenomena, focusing on molecular synthesis, modification, mechanisms and interactions. Thecentral dogma of molecular biology,where genetic material is transcribed into RNA and then translated intoprotein,despite being oversimplified, still provides a good starting point for understanding the field. This concept has been revised in light of emerging novel roles forRNA.
- Chemical biologyseeks to develop new tools based onsmall moleculesthat allow minimal perturbation of biological systems while providing detailed information about their function. Further, chemical biology employs biological systems to create non-natural hybrids between biomolecules and synthetic devices (for example emptiedviral capsidsthat can delivergene therapyordrug molecules).
See also
[edit]Lists
[edit]See also
[edit]- Astrobiology
- Biochemistry (journal)
- Biological Chemistry (journal)
- Biophysics
- Chemical ecology
- Computational biomodeling
- Dedicated bio-based chemical
- EC number
- Hypothetical types of biochemistry
- International Union of Biochemistry and Molecular Biology
- Metabolome
- Metabolomics
- Molecular biology
- Molecular medicine
- Plant biochemistry
- Proteolysis
- Small molecule
- Structural biology
- TCA cycle
Notes
[edit]- ^Fructose is not the only sugar found in fruits. Glucose and sucrose are also found in varying quantities in various fruits, and sometimes exceed the fructose present. For example, 32% of the edible portion of adateis glucose, compared with 24% fructose and 8% sucrose. However,peachescontain more sucrose (6.66%) than they do fructose (0.93%) or glucose (1.47%).[37]
References
[edit]- ^"Biological/Biochemistry".acs.org.Archivedfrom the original on 2019-08-21.Retrieved2016-01-04.
- ^abVoet(2005), p. 3.
- ^Karp(2009), p. 2.
- ^Miller(2012). p. 62.
- ^Astbury(1961), p. 1124.
- ^Eldra(2007), p. 45.
- ^Marks(2012), Chapter 14.
- ^Finkel(2009), pp. 1–4.
- ^UNICEF(2010), pp. 61, 75.
- ^abHelvoort(2000), p. 81.
- ^Hunter(2000), p. 75.
- ^Hamblin(2005), p. 26.
- ^Hunter(2000), pp. 96–98.
- ^Berg(1980), pp. 1–2.
- ^Holmes(1987), p. xv.
- ^Feldman(2001), p. 206.
- ^Rayner-Canham(2005), p. 136.
- ^Ziesak(1999), p. 169.
- ^Kleinkauf(1988), p. 116.
- ^Ben-Menahem(2009), p. 2982.
- ^Amsler(1986), p. 55.
- ^Horton(2013), p. 36.
- ^Kleinkauf(1988), p. 43.
- ^Edwards(1992), pp. 1161–1173.
- ^Fiske(1890), pp. 419–20.
- ^Wöhler, F. (1828)."Ueber künstliche Bildung des Harnstoffs".Annalen der Physik und Chemie.88(2): 253–256.Bibcode:1828AnP....88..253W.doi:10.1002/andp.18280880206.ISSN0003-3804.Archivedfrom the original on 2023-10-28.Retrieved2021-05-04.
- ^Kauffman(2001), pp. 121–133.
- ^Lipman, Timothy O. (August 1964)."Wohler's preparation of urea and the fate of vitalism".Journal of Chemical Education.41(8): 452.Bibcode:1964JChEd..41..452L.doi:10.1021/ed041p452.ISSN0021-9584.Archivedfrom the original on 2023-10-28.Retrieved2021-05-04.
- ^Tropp(2012), pp. 19–20.
- ^Krebs(2012), p. 32.
- ^Butler(2009), p. 5.
- ^Chandan(2007), pp. 193–194.
- ^Cox, Nelson, Lehninger (2008).Lehninger Principles of Biochemistry.Macmillan.
{{cite book}}
:CS1 maint: multiple names: authors list (link) - ^Nielsen(1999), pp. 283–303.
- ^Slabaugh(2007), pp. 3–6.
- ^Whiting(1970), pp. 1–31.
- ^Whiting,G.C. (1970), p. 5.
- ^Voet(2005), pp. 358–359.
- ^Varki(1999), p. 17.
- ^Stryer(2007), p. 328.
- ^Voet(2005), Ch. 12 Lipids and Membranes.
- ^Ahmed, Saba; Shah, Parini; Ahmed, Owais (2023),"Biochemistry, Lipids",StatPearls,Treasure Island (FL): StatPearls Publishing,PMID30247827,retrieved2023-11-30
- ^Metzler(2001), p. 58.
- ^Feige, Matthias J.; Hendershot, Linda M.; Buchner, Johannes (2010)."How antibodies fold".Trends in Biochemical Sciences.35(4): 189–198.doi:10.1016/j.tibs.2009.11.005.PMC4716677.PMID20022755.
- ^Fromm and Hargrove(2012), pp. 35–51.
- ^Saenger(1984), p. 84.
- ^"Disaccharide".Encyclopedia Britannica.Archivedfrom the original on 19 October 2023.Retrieved14 October2023.
- ^Fromm and Hargrove(2012), pp. 163–180.
- ^Voet(2005), Ch. 17 Glycolysis.
- ^A Dictionary of Biology.Oxford University Press. 17 September 2015.ISBN9780198714378.Archivedfrom the original on 10 July 2020.Retrieved29 April2020.
- ^Fromm and Hargrove(2012), pp. 183–194.
Cited literature
[edit]- Amsler, Mark (1986).The Languages of Creativity: Models, Problem-solving, Discourse.University of Delaware Press.ISBN978-0-87413-280-9.Archivedfrom the original on 2023-10-28.Retrieved2015-07-27.
- Astbury, W.T. (1961)."Molecular Biology or Ultrastructural Biology?".Nature.190(4781): 1124.Bibcode:1961Natur.190.1124A.doi:10.1038/1901124a0.PMID13684868.S2CID4172248.
- Ben-Menahem, Ari (2009).Historical Encyclopedia of Natural and Mathematical Sciences.Springer. p. 2982.Bibcode:2009henm.book.....B.ISBN978-3-540-68831-0.
- Burton, Feldman (2001).The Nobel Prize: A History of Genius, Controversy, and Prestige.Arcade Publishing.ISBN978-1-55970-592-9.
- Butler, John M. (2009).Fundamentals of Forensic DNA Typing.Academic Press.ISBN978-0-08-096176-7.
- Sen, Chandan K.; Roy, Sashwati (2007). "MiRNA: Licensed to Kill the Messenger".DNA and Cell Biology.26(4): 193–194.doi:10.1089/dna.2006.0567.PMID17465885.S2CID10665411.
- Clarence, Peter Berg (1980).The University of Iowa and Biochemistry from Their Beginnings.University of Iowa.ISBN978-0-87414-014-9.Archivedfrom the original on 2023-10-28.Retrieved2015-07-27.
- Edwards, Karen J.; Brown, David G.; Spink, Neil; Skelly, Jane V.; Neidle, Stephen (1992). "Molecular structure of the B-DNA dodecamer d(CGCAAATTTGCG)2 an examination of propeller twist and minor-groove water structure at 2·2Åresolution".Journal of Molecular Biology.226(4): 1161–1173.doi:10.1016/0022-2836(92)91059-x.PMID1518049.
- Eldra P. Solomon; Linda R. Berg; Diana W. Martin (2007).Biology, 8th Edition, International Student Edition.Thomson Brooks/Cole.ISBN978-0-495-31714-2.Archived fromthe originalon 2016-03-04.
- Fariselli, P.; Rossi, I.; Capriotti, E.; Casadio, R. (2006)."The WWWH of remote homolog detection: The state of the art".Briefings in Bioinformatics.8(2): 78–87.doi:10.1093/bib/bbl032.PMID17003074.
- Fiske, John (1890).Outlines of Cosmic Philosophy Based on the Doctrines of Evolution, with Criticisms on the Positive Philosophy, Volume 1.Boston and New York: Houghton, Mifflin.Archivedfrom the original on 28 October 2023.Retrieved16 February2015.
- Finkel, Richard; Cubeddu, Luigi; Clark, Michelle (2009).Lippincott's Illustrated Reviews: Pharmacology(4th ed.). Lippincott Williams & Wilkins.ISBN978-0-7817-7155-9.Archivedfrom the original on 2023-10-28.Retrieved2020-06-05.
- Krebs, Jocelyn E.; Goldstein, Elliott S.; Lewin, Benjamin; Kilpatrick, Stephen T. (2012).Essential Genes.Jones & Bartlett Publishers.ISBN978-1-4496-1265-8.
- Fromm, Herbert J.; Hargrove, Mark (2012).Essentials of Biochemistry.Springer.ISBN978-3-642-19623-2.Archivedfrom the original on 2023-10-28.Retrieved2020-06-05.
- Hamblin, Jacob Darwin (2005).Science in the Early Twentieth Century: An Encyclopedia.ABC-CLIO.ISBN978-1-85109-665-7.Archivedfrom the original on 2023-10-28.Retrieved2015-07-27.
- Helvoort, Ton van (2000). Arne Hessenbruch (ed.).Reader's Guide to the History of Science.Fitzroy Dearborn Publishing.ISBN978-1-884964-29-9.Archivedfrom the original on 2023-10-28.Retrieved2015-07-27.
- Holmes, Frederic Lawrence (1987).Lavoisier and the Chemistry of Life: An Exploration of Scientific Creativity.University of Wisconsin Press.ISBN978-0-299-09984-8.Archivedfrom the original on 2023-10-28.Retrieved2015-07-27.
- Horton, Derek, ed. (2013).Advances in Carbohydrate Chemistry and Biochemistry, Volume 70.Academic Press.ISBN978-0-12-408112-3.Archivedfrom the original on 2023-10-28.Retrieved2015-07-27.
- Hunter, Graeme K. (2000).Vital Forces: The Discovery of the Molecular Basis of Life.Academic Press.ISBN978-0-12-361811-5.Archivedfrom the original on 2023-10-28.Retrieved2020-06-05.
- Karp, Gerald (2009).Cell and Molecular Biology: Concepts and Experiments.John Wiley & Sons.ISBN978-0-470-48337-4.
- Kauffman, George B.; Chooljian, Steven H. (2001). "Friedrich Wöhler (1800–1882), on the Bicentennial of His Birth".The Chemical Educator.6(2): 121–133.doi:10.1007/s00897010444a.S2CID93425404.
- Kleinkauf, Horst; Döhren, Hans von; Jaenicke Lothar (1988).The Roots of Modern Biochemistry: Fritz Lippmann's Squiggle and its Consequences.Walter de Gruyter & Co. p. 116.ISBN978-3-11-085245-5.Archivedfrom the original on 2023-10-28.Retrieved2015-07-27.
- Knowles, J.R. (1980). "Enzyme-Catalyzed Phosphoryl Transfer Reactions".Annual Review of Biochemistry.49:877–919.doi:10.1146/annurev.bi.49.070180.004305.PMID6250450.S2CID7452392.
- Metzler, David Everett; Metzler, Carol M. (2001).Biochemistry: The Chemical Reactions of Living Cells.Vol. 1. Academic Press.ISBN978-0-12-492540-3.
- Miller G; Spoolman Scott (2012).Environmental Science – Biodiversity Is a Crucial Part of the Earth's Natural Capital.Cengage Learning.ISBN978-1-133-70787-5.Archivedfrom the original on 2023-04-13.Retrieved2016-01-04.
- Nielsen, Forrest H. (1999). "Ultratrace minerals". In Maurice E. Shils; et al. (eds.).Modern Nutrition in Health and Disease.Baltimore: Williams & Wilkins. pp. 283–303.hdl:10113/46493.
- Peet, Alisa (2012). Marks, Allan; Lieberman Michael A. (eds.).Marks' Basic Medical Biochemistry (Lieberman, Marks's Basic Medical Biochemistry)(4th ed.). Lippincott Williams & Wilkins.ISBN978-1-60831-572-7.Archivedfrom the original on 2023-10-28.Retrieved2020-06-05.
- Rayner-Canham, Marelene F.; Rayner-Canham, Marelene; Rayner-Canham, Geoffrey (2005).Women in Chemistry: Their Changing Roles from Alchemical Times to the Mid-Twentieth Century.Chemical Heritage Foundation.ISBN978-0-941901-27-7.
- Rojas-Ruiz, Fernando A.; Vargas-Méndez, Leonor Y.; Kouznetsov, Vladimir V. (2011)."Challenges and Perspectives of Chemical Biology, a Successful Multidisciplinary Field of Natural Sciences".Molecules.16(3): 2672–2687.doi:10.3390/molecules16032672.PMC6259834.PMID21441869.
- Saenger, Wolfram (1984).Principles of Nucleic Acid Structure.New York: Springer-Verlag.ISBN978-0-387-90762-8.Archivedfrom the original on 2023-10-28.Retrieved2020-06-05.
- Slabaugh, Michael R.; Seager, Spencer L. (2013).Organic and Biochemistry for Today(6th ed.). Pacific Grove: Brooks Cole.ISBN978-1-133-60514-0.Archivedfrom the original on 2023-10-28.Retrieved2020-06-05.
- Sherwood, Lauralee; Klandorf, Hillar; Yancey, Paul H. (2012).Animal Physiology: From Genes to Organisms.Cengage Learning.ISBN978-0-8400-6865-1.
- Stryer L, Berg JM, Tymoczko JL (2007).Biochemistry(6th ed.). San Francisco: W.H. Freeman.ISBN978-0-7167-8724-2.
- Tropp, Burton E. (2012).Molecular Biology(4th ed.). Jones & Bartlett Learning.ISBN978-1-4496-0091-4.Archivedfrom the original on 2023-10-28.Retrieved2020-06-05.
- UNICEF (2010).Facts for life(PDF)(4th ed.). New York: United Nations Children's Fund.ISBN978-92-806-4466-1.Archived(PDF)from the original on 2022-10-09.
- Ulveling, Damien; Francastel, Claire; Hubé, Florent (2011)."When one is better than two: RNA with dual functions"(PDF).Biochimie.93(4): 633–644.doi:10.1016/j.biochi.2010.11.004.PMID21111023.S2CID22165949.Archived(PDF)from the original on 2022-10-09.
- Varki A, Cummings R, Esko J, Jessica F, Hart G, Marth J (1999).Essentials of glycobiology.Cold Spring Harbor Laboratory Press.ISBN978-0-87969-560-6.Archivedfrom the original on 2023-10-28.Retrieved2020-06-05.
- Voet, D; Voet, JG (2005).Biochemistry(3rd ed.). Hoboken, NJ: John Wiley & Sons Inc.ISBN978-0-471-19350-0.Archived fromthe originalon September 11, 2007.
- Whiting, G.C (1970)."Sugars".In A.C. Hulme (ed.).The Biochemistry of Fruits and their Products.Vol. 1. London & New York: Academic Press.ISBN978-0-12-361201-4.
- Ziesak, Anne-Katrin; Cram Hans-Robert (1999).Walter de Gruyter Publishers, 1749–1999.Walter de Gruyter & Co.ISBN978-3-11-016741-2.Archivedfrom the original on 2023-10-28.Retrieved2015-07-27.
- Ashcroft, Steve. "Professor Sir Philip Randle; Researcher into metabolism: [1st Edition]".Independent.ProQuest311080685.
Further reading
[edit]- Fruton, Joseph S.Proteins, Enzymes, Genes: The Interplay of Chemistry and Biology.Yale University Press: New Haven, 1999.ISBN0-300-07608-8
- Keith Roberts, Martin Raff, Bruce Alberts, Peter Walter, Julian Lewis and Alexander Johnson,Molecular Biology of the Cell
- 4th Edition, Routledge, March, 2002, hardcover, 1616 pp.ISBN0-8153-3218-1
- 3rd Edition, Garland, 1994,ISBN0-8153-1620-8
- 2nd Edition, Garland, 1989,ISBN0-8240-3695-6
- Kohler, Robert.From Medical Chemistry to Biochemistry: The Making of a Biomedical Discipline.Cambridge University Press, 1982.
- Maggio, Lauren A.; Willinsky, John M.; Steinberg, Ryan M.; Mietchen, Daniel; Wass, Joseph L.; Dong, Ting (2017)."Wikipedia as a gateway to biomedical research: The relative distribution and use of citations in the English Wikipedia".PLOS ONE.12(12): e0190046.Bibcode:2017PLoSO..1290046M.doi:10.1371/journal.pone.0190046.PMC5739466.PMID29267345.
External links
[edit]


- "Biochemical Society".
- The Virtual Library of Biochemistry, Molecular Biology and Cell Biology
- Biochemistry, 5th ed.Full text of Berg, Tymoczko, and Stryer, courtesy ofNCBI.
- SystemsX.ch – The Swiss Initiative in Systems Biology
- Full text of Biochemistryby Kevin and Indira, an introductory biochemistry textbook.