Condensed matter physics
Condensed matter physics |
---|
![]() |
Condensed matter physicsis the field ofphysicsthat deals with the macroscopic and microscopic physical properties ofmatter,especially thesolidandliquidphases,that arise fromelectromagneticforces betweenatomsandelectrons.More generally, the subject deals with condensed phases of matter: systems of many constituents with strong interactions among them. More exotic condensed phases include thesuperconductingphase exhibited by certain materials at extremely lowcryogenictemperatures,theferromagneticandantiferromagneticphases ofspinsoncrystal latticesof atoms, theBose–Einstein condensatesfound inultracold atomicsystems, andliquid crystals.Condensed matter physicists seek to understand the behavior of these phases by experiments to measure various material properties, and by applying thephysical lawsofquantum mechanics,electromagnetism,statistical mechanics,and otherphysics theoriesto develop mathematical models and predict the properties of extremely large groups of atoms.[1]
The diversity of systems and phenomena available for study makes condensed matter physics the most active field of contemporary physics: one third of all American physicists self-identify as condensed matter physicists,[2]and the Division of Condensed Matter Physics is the largest division of theAmerican Physical Society.[3]These include solid state andsoft matterphysicists, who studyquantumand non-quantum physical properties of matter respectively.[4]Both types study a great range of materials, providing many research, funding and employment opportunities.[5]The field overlaps withchemistry,materials science,engineeringandnanotechnology,and relates closely toatomic physicsandbiophysics.Thetheoretical physicsof condensed matter shares important concepts and methods with that ofparticle physicsandnuclear physics.[6]
A variety of topics in physics such ascrystallography,metallurgy,elasticity,magnetism,etc., were treated as distinct areas until the 1940s, when they were grouped together assolid-state physics.Around the 1960s, the study of physical properties ofliquidswas added to this list, forming the basis for the more comprehensive specialty of condensed matter physics.[7]TheBell Telephone Laboratorieswas one of the first institutes to conduct a research program in condensed matter physics.[7]According to the founding director of theMax Planck Institute for Solid State Research,physics professor Manuel Cardona, it wasAlbert Einsteinwho created the modern field of condensed matter physics starting with his seminal 1905 article on thephotoelectric effectandphotoluminescencewhich opened the fields ofphotoelectron spectroscopyandphotoluminescence spectroscopy,and later his 1907 article on thespecific heat of solidswhich introduced, for the first time, the effect of lattice vibrations on the thermodynamic properties of crystals, in particular thespecific heat.[8]Deputy Director of the Yale Quantum InstituteA. Douglas Stonemakes a similar priority case for Einstein in his work on the synthetic history ofquantum mechanics.[9]
Etymology
[edit]According to physicistPhilip Warren Anderson,the use of the term "condensed matter" to designate a field of study was coined by him andVolker Heine,when they changed the name of their group at theCavendish Laboratories,Cambridge,fromSolid state theorytoTheory of Condensed Matterin 1967,[10]as they felt it better included their interest in liquids,nuclear matter,and so on.[11][12]Although Anderson and Heine helped popularize the name "condensed matter", it had been used in Europe for some years, most prominently in theSpringer-VerlagjournalPhysics of Condensed Matter,launched in 1963.[13]The name "condensed matter physics" emphasized the commonality of scientific problems encountered by physicists working on solids, liquids, plasmas, and other complex matter, whereas "solid state physics" was often associated with restricted industrial applications of metals and semiconductors. In the 1960s and 70s, some physicists felt the more comprehensive name better fit the funding environment andCold Warpolitics of the time.[14]
References to "condensed" states can be traced to earlier sources. For example, in the introduction to his 1947 bookKinetic Theory of Liquids,[15]Yakov Frenkelproposed that "The kinetic theory of liquids must accordingly be developed as a generalization and extension of the kinetic theory of solid bodies. As a matter of fact, it would be more correct to unify them under the title of 'condensed bodies'".
History
[edit]Classical physics
[edit]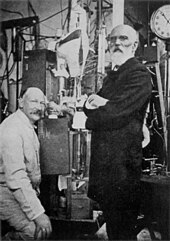
One of the first studies of condensed states of matter was byEnglishchemistHumphry Davy,in the first decades of the nineteenth century. Davy observed that of the fortychemical elementsknown at the time, twenty-six hadmetallicproperties such aslustre,ductilityand high electrical and thermal conductivity.[16]This indicated that the atoms inJohn Dalton'satomic theorywere not indivisible as Dalton claimed, but had inner structure. Davy further claimed that elements that were then believed to be gases, such asnitrogenandhydrogencould be liquefied under the right conditions and would then behave as metals.[17][note 1]
In 1823,Michael Faraday,then an assistant in Davy's lab, successfully liquefiedchlorineand went on to liquefy all known gaseous elements, except for nitrogen, hydrogen, andoxygen.[16]Shortly after, in 1869,IrishchemistThomas Andrewsstudied thephase transitionfrom a liquid to a gas and coined the termcritical pointto describe the condition where a gas and a liquid were indistinguishable as phases,[19]andDutchphysicistJohannes van der Waalssupplied the theoretical framework which allowed the prediction of critical behavior based on measurements at much higher temperatures.[20]: 35–38 By 1908,James DewarandHeike Kamerlingh Onneswere successfully able to liquefy hydrogen and the then newly discoveredheliumrespectively.[16]
Paul Drudein 1900 proposed the first theoretical model for a classicalelectronmoving through a metallic solid.[6]Drude's model described properties of metals in terms of a gas of free electrons, and was the first microscopic model to explain empirical observations such as theWiedemann–Franz law.[21][22]: 27–29 However, despite the success ofDrude's model,it had one notable problem: it was unable to correctly explain the electronic contribution to thespecific heatand magnetic properties of metals, and the temperature dependence of resistivity at low temperatures.[23]: 366–368
In 1911, three years after helium was first liquefied, Onnes working atUniversity of Leidendiscoveredsuperconductivityinmercury,when he observed the electrical resistivity of mercury to vanish at temperatures below a certain value.[24]The phenomenon completely surprised the best theoretical physicists of the time, and it remained unexplained for several decades.[25]Albert Einstein,in 1922, said regarding contemporary theories of superconductivity that "with our far-reaching ignorance of the quantum mechanics of composite systems we are very far from being able to compose a theory out of these vague ideas."[26]
Advent of quantum mechanics
[edit]Drude's classical model was augmented byWolfgang Pauli,Arnold Sommerfeld,Felix Blochand other physicists. Pauli realized that the free electrons in metal must obey theFermi–Dirac statistics.Using this idea, he developed the theory ofparamagnetismin 1926. Shortly after, Sommerfeld incorporated theFermi–Dirac statisticsinto thefree electron modeland made it better to explain the heat capacity. Two years later, Bloch usedquantum mechanicsto describe the motion of an electron in a periodic lattice.[23]: 366–368
The mathematics of crystal structures developed byAuguste Bravais,Yevgraf Fyodorovand others was used to classify crystals by theirsymmetry group,and tables of crystal structures were the basis for the seriesInternational Tables of Crystallography,first published in 1935.[27]Band structure calculationswere first used in 1930 to predict the properties of new materials, and in 1947John Bardeen,Walter BrattainandWilliam Shockleydeveloped the firstsemiconductor-basedtransistor,heralding a revolution in electronics.[6]

In 1879,Edwin Herbert Hallworking at theJohns Hopkins Universitydiscovered that a voltage developed across conductors which was transverse to both an electric current in the conductor and a magnetic field applied perpendicular to the current.[28]This phenomenon, arising due to the nature of charge carriers in the conductor, came to be termed theHall effect,but it was not properly explained at the time because the electron was not experimentally discovered until 18 years later. After the advent of quantum mechanics,Lev Landauin 1930 developed the theory ofLandau quantizationand laid the foundation for a theoretical explanation of thequantum Hall effectwhich was discovered half a century later.[29]: 458–460 [30]
Magnetism as a property of matter has been known in China since 4000 BC.[31]: 1–2 However, the first modern studies of magnetism only started with the development ofelectrodynamicsby Faraday,Maxwelland others in the nineteenth century, which included classifying materials asferromagnetic,paramagneticanddiamagneticbased on their response to magnetization.[32]Pierre Curiestudied the dependence of magnetization on temperature and discovered theCurie pointphase transition in ferromagnetic materials.[31]In 1906,Pierre Weissintroduced the concept ofmagnetic domainsto explain the main properties of ferromagnets.[33]: 9 The first attempt at a microscopic description of magnetism was byWilhelm LenzandErnst Isingthrough theIsing modelthat described magnetic materials as consisting of a periodic lattice ofspinsthat collectively acquired magnetization.[31]The Ising model was solved exactly to show thatspontaneous magnetizationcan occur in one dimension and it is possible in higher-dimensional lattices. Further research such as by Bloch onspin wavesandNéelonantiferromagnetismled to developing new magnetic materials with applications tomagnetic storagedevices.[31]: 36–38, g48
Modern many-body physics
[edit]
The Sommerfeld model and spin models for ferromagnetism illustrated the successful application of quantum mechanics to condensed matter problems in the 1930s. However, there still were several unsolved problems, most notably the description ofsuperconductivityand theKondo effect.[35]AfterWorld War II,several ideas from quantum field theory were applied to condensed matter problems. These included recognition ofcollective excitationmodes of solids and the important notion of a quasiparticle. Soviet physicistLev Landauused the idea for theFermi liquid theorywherein low energy properties of interacting fermion systems were given in terms of what are now termed Landau-quasiparticles.[35]Landau also developed amean-field theoryfor continuous phase transitions, which described ordered phases asspontaneous breakdown of symmetry.The theory also introduced the notion of anorder parameterto distinguish between ordered phases.[36]Eventually in 1956,John Bardeen,Leon CooperandRobert Schriefferdeveloped the so-calledBCS theoryof superconductivity, based on the discovery that arbitrarily small attraction between two electrons of opposite spin mediated byphononsin the lattice can give rise to a bound state called aCooper pair.[37]

The study of phase transitions and the critical behavior of observables, termedcritical phenomena,was a major field of interest in the 1960s.[39]Leo Kadanoff,Benjamin WidomandMichael Fisherdeveloped the ideas ofcritical exponentsandwidom scaling.These ideas were unified byKenneth G. Wilsonin 1972, under the formalism of therenormalization groupin the context of quantum field theory.[39]
Thequantum Hall effectwas discovered byKlaus von Klitzing,Dorda and Pepper in 1980 when they observed the Hall conductance to be integer multiples of a fundamental constant.(see figure) The effect was observed to be independent of parameters such as system size and impurities.[38]In 1981, theoristRobert Laughlinproposed a theory explaining the unanticipated precision of the integral plateau. It also implied that the Hall conductance is proportional to a topological invariant, calledChern number,whose relevance for the band structure of solids was formulated byDavid J. Thoulessand collaborators.[40][41]: 69, 74 Shortly after, in 1982,Horst StörmerandDaniel Tsuiobserved thefractional quantum Hall effectwhere the conductance was now a rational multiple of the constant.Laughlin, in 1983, realized that this was a consequence of quasiparticle interaction in the Hall states and formulated avariational methodsolution, named theLaughlin wavefunction.[42]The study of topological properties of the fractional Hall effect remains an active field of research.[43]Decades later, the aforementioned topological band theory advanced byDavid J. Thoulessand collaborators[44]was further expanded leading to the discovery oftopological insulators.[45][46]
In 1986,Karl MüllerandJohannes Bednorzdiscovered the firsthigh temperature superconductor,La2-xBaxCuO4,which is superconducting at temperatures as high as 39kelvin.[47]It was realized that the high temperature superconductors are examples of strongly correlated materials where the electron–electron interactions play an important role.[48]A satisfactory theoretical description of high-temperature superconductors is still not known and the field ofstrongly correlated materialscontinues to be an active research topic.
In 2012, several groups released preprints which suggest thatsamarium hexaboridehas the properties of atopological insulator[49]in accord with the earlier theoretical predictions.[50]Since samarium hexaboride is an establishedKondo insulator,i.e. a strongly correlated electron material, it is expected that the existence of a topological Dirac surface state in this material would lead to a topological insulator with strong electronic correlations.
Theoretical
[edit]Theoretical condensed matter physics involves the use of theoretical models to understand properties of states of matter. These include models to study the electronic properties of solids, such as theDrude model,theband structureand thedensity functional theory.Theoretical models have also been developed to study the physics ofphase transitions,such as theGinzburg–Landau theory,critical exponentsand the use of mathematical methods ofquantum field theoryand therenormalization group.Modern theoretical studies involve the use ofnumerical computationof electronic structure and mathematical tools to understand phenomena such ashigh-temperature superconductivity,topological phases,andgauge symmetries.
Emergence
[edit]Theoretical understanding of condensed matter physics is closely related to the notion ofemergence,wherein complex assemblies of particles behave in ways dramatically different from their individual constituents.[37][43]For example, a range of phenomena related to high temperature superconductivity are understood poorly, although the microscopic physics of individual electrons and lattices is well known.[51]Similarly, models of condensed matter systems have been studied wherecollective excitationsbehave likephotonsandelectrons,thereby describingelectromagnetismas an emergent phenomenon.[52]Emergent properties can also occur at the interface between materials: one example is thelanthanum aluminate-strontium titanate interface,where two band-insulators are joined to create conductivity andsuperconductivity.
Electronic theory of solids
[edit]The metallic state has historically been an important building block for studying properties of solids.[53]The first theoretical description of metals was given byPaul Drudein 1900 with theDrude model,which explained electrical and thermal properties by describing a metal as anideal gasof then-newly discoveredelectrons.He was able to derive the empiricalWiedemann-Franz lawand get results in close agreement with the experiments.[22]: 90–91 This classical model was then improved byArnold Sommerfeldwho incorporated theFermi–Dirac statisticsof electrons and was able to explain the anomalous behavior of thespecific heatof metals in theWiedemann–Franz law.[22]: 101–103 In 1912, The structure of crystalline solids was studied byMax von Laueand Paul Knipping, when they observed theX-ray diffractionpattern of crystals, and concluded that crystals get their structure from periodiclatticesof atoms.[22]: 48 [54]In 1928, Swiss physicistFelix Blochprovided a wave function solution to theSchrödinger equationwith aperiodicpotential, known asBloch's theorem.[55]
Calculating electronic properties of metals by solving the many-body wavefunction is often computationally hard, and hence, approximation methods are needed to obtain meaningful predictions.[56]TheThomas–Fermi theory,developed in the 1920s, was used to estimate system energy and electronic density by treating the local electron density as avariational parameter.Later in the 1930s,Douglas Hartree,Vladimir FockandJohn Slaterdeveloped the so-calledHartree–Fock wavefunctionas an improvement over the Thomas–Fermi model. The Hartree–Fock method accounted forexchange statisticsof single particle electron wavefunctions. In general, it is very difficult to solve the Hartree–Fock equation. Only the free electron gas case can be solved exactly.[53]: 330–337 Finally in 1964–65,Walter Kohn,Pierre HohenbergandLu Jeu Shamproposed thedensity functional theory(DFT) which gave realistic descriptions for bulk and surface properties of metals. The density functional theory has been widely used since the 1970s for band structure calculations of variety of solids.[56]
Symmetry breaking
[edit]Some states of matter exhibitsymmetry breaking,where the relevant laws of physics possess some form ofsymmetrythat is broken. A common example iscrystalline solids,which break continuoustranslational symmetry.Other examples include magnetizedferromagnets,which breakrotational symmetry,and more exotic states such as the ground state of aBCSsuperconductor,that breaksU(1)phase rotational symmetry.[57][58]
Goldstone's theoreminquantum field theorystates that in a system with broken continuous symmetry, there may exist excitations with arbitrarily low energy, called the Goldstonebosons.For example, in crystalline solids, these correspond tophonons,which are quantized versions of lattice vibrations.[59]
Phase transition
[edit]Phase transition refers to the change of phase of a system, which is brought about by change in an external parameter such astemperature,pressure,ormolar composition.In a single-component system, a classical phase transition occurs at a temperature (at a specific pressure) where there is an abrupt change in the order of the system For example, when ice melts and becomes water, the ordered hexagonal crystal structure of ice is modified to a hydrogen bonded, mobile arrangement of water molecules.
Inquantum phase transitions,the temperature is set toabsolute zero,and the non-thermal control parameter, such as pressure or magnetic field, causes the phase transitions when order is destroyed byquantum fluctuationsoriginating from theHeisenberg uncertainty principle.Here, the different quantum phases of the system refer to distinctground statesof theHamiltonian matrix.Understanding the behavior of quantum phase transition is important in the difficult tasks of explaining the properties of rare-earth magnetic insulators, high-temperature superconductors, and other substances.[60]
Two classes of phase transitions occur:first-order transitionsandsecond-orderorcontinuous transitions.For the latter, the two phases involved do not co-exist at the transition temperature, also called thecritical point.Near the critical point, systems undergo critical behavior, wherein several of their properties such ascorrelation length,specific heat,andmagnetic susceptibilitydiverge exponentially.[60]These critical phenomena present serious challenges to physicists because normalmacroscopiclaws are no longer valid in the region, and novel ideas and methods must be invented to find the new laws that can describe the system.[61]: 75ff
The simplest theory that can describe continuous phase transitions is theGinzburg–Landau theory,which works in the so-calledmean-field approximation.However, it can only roughly explain continuous phase transition for ferroelectrics and type I superconductors which involves long range microscopic interactions. For other types of systems that involves short range interactions near the critical point, a better theory is needed.[62]: 8–11
Near the critical point, the fluctuations happen over broad range of size scales while the feature of the whole system is scale invariant.Renormalization groupmethods successively average out the shortest wavelength fluctuations in stages while retaining their effects into the next stage. Thus, the changes of a physical system as viewed at different size scales can be investigated systematically. The methods, together with powerful computer simulation, contribute greatly to the explanation of the critical phenomena associated with continuous phase transition.[61]: 11
Experimental
[edit]Experimental condensed matter physics involves the use of experimental probes to try to discover new properties of materials. Such probes include effects ofelectricandmagnetic fields,measuringresponse functions,transport propertiesandthermometry.[63]Commonly used experimental methods includespectroscopy,with probes such asX-rays,infrared lightandinelastic neutron scattering;study of thermal response, such asspecific heatand measuring transport via thermal and heatconduction.

Scattering
[edit]Several condensed matter experiments involve scattering of an experimental probe, such asX-ray,opticalphotons,neutrons,etc., on constituents of a material. The choice of scattering probe depends on the observation energy scale of interest.Visible lighthas energy on the scale of 1electron volt(eV) and is used as a scattering probe to measure variations in material properties such as thedielectric constantandrefractive index.X-rays have energies of the order of 10keVand hence are able to probe atomic length scales, and are used to measure variations in electron charge density and crystal structure.[64]: 33–34
Neutronscan also probe atomic length scales and are used to study the scattering off nuclei and electronspinsand magnetization (as neutrons have spin but no charge). Coulomb andMott scatteringmeasurements can be made by usingelectron beamsas scattering probes.[64]: 33–34 [65]: 39–43 Similarly,positronannihilation can be used as an indirect measurement of local electron density.[66]Laser spectroscopyis an excellent tool for studying the microscopic properties of a medium, for example, to studyforbidden transitionsin media withnonlinear optical spectroscopy.[61]: 258–259
External magnetic fields
[edit]In experimental condensed matter physics, externalmagnetic fieldsact asthermodynamic variablesthat control the state, phase transitions and properties of material systems.[67]Nuclear magnetic resonance(NMR) is a method by which externalmagnetic fieldsare used to find resonance modes of individual nuclei, thus giving information about the atomic, molecular, and bond structure of their environment. NMR experiments can be made in magnetic fields with strengths up to 60tesla.Higher magnetic fields can improve the quality of NMR measurement data.[68]: 69 [69]: 185 Quantum oscillationsis another experimental method where high magnetic fields are used to study material properties such as the geometry of theFermi surface.[70]High magnetic fields will be useful in experimental testing of the various theoretical predictions such as the quantizedmagnetoelectric effect,imagemagnetic monopole,and the half-integerquantum Hall effect.[68]: 57
Magnetic resonance spectroscopy
[edit]Thelocal structure,as well as the structure of the nearest neighbour atoms, can be investigated in condensed matter with magnetic resonance methods, such aselectron paramagnetic resonance(EPR) andnuclear magnetic resonance(NMR), which are very sensitive to the details of the surrounding of nuclei and electrons by means of the hyperfine coupling. Both localized electrons and specific stable or unstable isotopes of thenucleibecome the probe of thesehyperfine interactions), which couple the electron or nuclear spin to the local electric and magnetic fields. These methods are suitable to study defects, diffusion, phase transitions and magnetic order. Common experimental methods includeNMR,nuclear quadrupole resonance(NQR), implanted radioactive probes as in the case ofmuon spin spectroscopy(SR),Mössbauer spectroscopy,NMR andperturbed angular correlation(PAC). PAC is especially ideal for the study of phase changes at extreme temperatures above 2000 °C due to the temperature independence of the method.
Cold atomic gases
[edit]
Ultracold atomtrapping in optical lattices is an experimental tool commonly used in condensed matter physics, and inatomic, molecular, and optical physics.The method involves using optical lasers to form aninterference pattern,which acts as alattice,in which ions or atoms can be placed at very low temperatures. Cold atoms in optical lattices are used asquantum simulators,that is, they act as controllable systems that can model behavior of more complicated systems, such asfrustrated magnets.[71]In particular, they are used to engineer one-, two- and three-dimensional lattices for aHubbard modelwith pre-specified parameters, and to study phase transitions forantiferromagneticandspin liquidordering.[72][73][43]
In 1995, a gas ofrubidiumatoms cooled down to a temperature of 170nKwas used to experimentally realize theBose–Einstein condensate,a novel state of matter originally predicted byS. N. BoseandAlbert Einstein,wherein a large number of atoms occupy onequantum state.[74]
Applications
[edit]
Research in condensed matter physics[43][75]has given rise to several device applications, such as the development of thesemiconductortransistor,[6]lasertechnology,[61]magnetic storage,liquid crystals,optical fibres[76]and several phenomena studied in the context ofnanotechnology.[77]: 111ff Methods such asscanning-tunneling microscopycan be used to control processes at thenanometerscale, and have given rise to the study of nanofabrication.[78]Such molecular machines were developed for example by Nobel laureates in chemistryBen Feringa,Jean-Pierre SauvageandFraser Stoddart.Feringa and his team developed multiple molecular machines such as themolecular car,molecular windmill and many more.[79]
Inquantum computation,information is represented by quantum bits, orqubits.The qubits maydecoherequickly before useful computation is completed. This serious problem must be solved before quantum computing may be realized. To solve this problem, several promising approaches are proposed in condensed matter physics, includingJosephson junctionqubits,spintronicqubits using thespinorientation of magnetic materials, and the topological non-Abeliananyonsfromfractional quantum Hall effectstates.[78]
Condensed matter physics also has important uses forbiomedicine,for example, the experimental method ofmagnetic resonance imaging,which is widely used in medical diagnosis.[78]
See also
[edit]- Soft matter– Subfield of condensed matter physics
- Green–Kubo relations– Equation relating transport coefficients to correlation functions
- Green's function (many-body theory)– Correlators of field operators
- Materials science– Research of materials
- Nuclear spectroscopy– Using nucleus properties to probe material properties
- Comparison of software for molecular mechanics modeling
- Transparent materials– Property of an object or substance to transmit light with minimal scattering
- Orbital magnetization
- Symmetry in quantum mechanics– Properties underlying modern physics
- Mesoscopic physics– Subdiscipline of condensed matter physics that deals with materials of an intermediate size
Notes
[edit]- ^Both hydrogen and nitrogen have since been liquified; however, ordinary liquid nitrogen and hydrogen do not possess metallic properties. PhysicistsEugene WignerandHillard Bell Huntingtonpredicted in 1935[18]that a statemetallic hydrogenexists at sufficiently high pressures (over 25GPa), but this has not yet been observed.
References
[edit]- ^"Condensed Matter Physics Theory".Yale University Physics Department.Retrieved2023-11-30.
- ^"Condensed Matter Physics Jobs: Careers in Condensed Matter Physics".Physics Today Jobs.Archived fromthe originalon 2009-03-27.Retrieved2010-11-01.
- ^"History of Condensed Matter Physics".American Physical Society.Retrieved27 March2012.
- ^"Condensed Matter Physics".University of Colorado Boulder Physics Department.26 April 2016.Retrieved2023-11-30.
- ^"Condensed Matter and Materials Physics".Iowa College of Liberal Arts and Sciences.Retrieved2023-11-30.
- ^abcdCohen, Marvin L. (2008)."Essay: Fifty Years of Condensed Matter Physics".Physical Review Letters.101(25): 250001.Bibcode:2008PhRvL.101y0001C.doi:10.1103/PhysRevLett.101.250001.PMID19113681.Retrieved31 March2012.
- ^abKohn, W. (1999)."An essay on condensed matter physics in the twentieth century"(PDF).Reviews of Modern Physics.71(2): S59–S77.Bibcode:1999RvMPS..71...59K.doi:10.1103/RevModPhys.71.S59.Archived fromthe original(PDF)on 25 August 2013.Retrieved27 March2012.
- ^Cardona, Manuel (31 August 2005). "Einstein as the Father of Solid State Physics".arXiv:physics/0508237.
- ^Stone, A. Douglas (6 October 2013).Einstein and the Quantum: The Quest of the Valiant Swabian(First ed.). Princeton University Press.ISBN978-0691139685.Retrieved1 June2022.
- ^"Philip Anderson".Department of Physics.Princeton University.Retrieved27 March2012.
- ^Anderson, Philip W. (November 2011)."In Focus: More and Different".World Scientific Newsletter.33:2.
- ^Anderson, Philip W. (2018-03-09).Basic Notions Of Condensed Matter Physics.CRC Press.ISBN978-0-429-97374-1.
- ^"Physics of Condensed Matter".1963.Retrieved20 April2015.
- ^Martin, Joseph D. (2015)."What's in a Name Change? Solid State Physics, Condensed Matter Physics, and Materials Science"(PDF).Physics in Perspective.17(1): 3–32.Bibcode:2015PhP....17....3M.doi:10.1007/s00016-014-0151-7.S2CID117809375.Archived(PDF)from the original on 2022-10-09.
- ^Frenkel, J. (1947).Kinetic Theory of Liquids.Oxford University Press.
- ^abcGoodstein, David;Goodstein, Judith(2000)."Richard Feynman and the History of Superconductivity"(PDF).Physics in Perspective.2(1): 30.Bibcode:2000PhP.....2...30G.doi:10.1007/s000160050035.S2CID118288008.Archived fromthe original(PDF)on 17 November 2015.Retrieved7 April2012.
- ^Davy, John, ed. (1839).The collected works of Sir Humphry Davy: Vol. II.Smith Elder & Co., Cornhill. p.22.
- ^Silvera, Isaac F.; Cole, John W. (2010)."Metallic Hydrogen: The Most Powerful Rocket Fuel Yet to Exist".Journal of Physics.215(1): 012194.Bibcode:2010JPhCS.215a2194S.doi:10.1088/1742-6596/215/1/012194.
- ^Rowlinson, J. S. (1969). "Thomas Andrews and the Critical Point".Nature.224(8): 541–543.Bibcode:1969Natur.224..541R.doi:10.1038/224541a0.S2CID4168392.
- ^Atkins, Peter; de Paula, Julio (2009).Elements of Physical Chemistry.Oxford University Press.ISBN978-1-4292-1813-9.
- ^Kittel, Charles (1996).Introduction to Solid State Physics.John Wiley & Sons.ISBN978-0-471-11181-8.
- ^abcdHoddeson, Lillian (1992).Out of the Crystal Maze: Chapters from The History of Solid State Physics.Oxford University Press.ISBN978-0-19-505329-6.
- ^abKragh, Helge (2002).Quantum Generations: A History of Physics in the Twentieth Century(Reprint ed.). Princeton University Press.ISBN978-0-691-09552-3.
- ^van Delft, Dirk; Kes, Peter (September 2010)."The discovery of superconductivity"(PDF).Physics Today.63(9): 38–43.Bibcode:2010PhT....63i..38V.doi:10.1063/1.3490499.Archived(PDF)from the original on 2022-10-09.Retrieved7 April2012.
- ^Slichter, Charles."Introduction to the History of Superconductivity".Moments of Discovery.American Institute of Physics. Archived fromthe originalon 15 May 2012.Retrieved13 June2012.
- ^Schmalian, Joerg (2010). "Failed theories of superconductivity".Modern Physics Letters B.24(27): 2679–2691.arXiv:1008.0447.Bibcode:2010MPLB...24.2679S.doi:10.1142/S0217984910025280.S2CID119220454.
- ^Aroyo, Mois, I.; Müller, Ulrich; Wondratschek, Hans (2006).Historical introduction(PDF).International Tables for Crystallography. Vol. A. pp. 2–5.CiteSeerX10.1.1.471.4170.doi:10.1107/97809553602060000537.ISBN978-1-4020-2355-2.Archived fromthe original(PDF)on 2008-10-03.Retrieved2017-10-24.
{{cite book}}
:CS1 maint: multiple names: authors list (link) - ^Hall, Edwin (1879)."On a New Action of the Magnet on Electric Currents".American Journal of Mathematics.2(3): 287–92.doi:10.2307/2369245.JSTOR2369245.S2CID107500183.Archived fromthe originalon 2007-02-08.Retrieved2008-02-28.
- ^Landau, L. D.; Lifshitz, E. M. (1977).Quantum Mechanics: Nonrelativistic Theory.Pergamon Press.ISBN978-0-7506-3539-4.
- ^Lindley, David (2015-05-15). "Focus: Landmarks—Accidental Discovery Leads to Calibration Standard".Physics.8:46.doi:10.1103/Physics.8.46.
- ^abcdMattis, Daniel (2006).The Theory of Magnetism Made Simple.World Scientific.ISBN978-981-238-671-7.
- ^Chatterjee, Sabyasachi (August 2004)."Heisenberg and Ferromagnetism".Resonance.9(8): 57–66.doi:10.1007/BF02837578.S2CID123099296.Retrieved13 June2012.
- ^Visintin, Augusto (1994).Differential Models of Hysteresis.Springer.ISBN978-3-540-54793-8.
- ^Merali, Zeeya (2011)."Collaborative physics: string theory finds a bench mate".Nature.478(7369): 302–304.Bibcode:2011Natur.478..302M.doi:10.1038/478302a.PMID22012369.
- ^abColeman, Piers (2003). "Many-Body Physics: Unfinished Revolution".Annales Henri Poincaré.4(2): 559–580.arXiv:cond-mat/0307004.Bibcode:2003AnHP....4..559C.CiteSeerX10.1.1.242.6214.doi:10.1007/s00023-003-0943-9.S2CID8171617.
- ^Kadanoff, Leo, P. (2009).Phases of Matter and Phase Transitions; From Mean Field Theory to Critical Phenomena(PDF).The University of Chicago. Archived fromthe original(PDF)on 2015-12-31.Retrieved2012-06-14.
{{cite book}}
:CS1 maint: multiple names: authors list (link) - ^abColeman, Piers (2016).Introduction to Many Body Physics.Cambridge University Press.ISBN978-0-521-86488-6.
- ^abvon Klitzing, Klaus (9 Dec 1985)."The Quantized Hall Effect"(PDF).Nobelprize.org.Archived(PDF)from the original on 2022-10-09.
- ^abFisher, Michael E. (1998). "Renormalization group theory: Its basis and formulation in statistical physics".Reviews of Modern Physics.70(2): 653–681.Bibcode:1998RvMP...70..653F.CiteSeerX10.1.1.129.3194.doi:10.1103/RevModPhys.70.653.
- ^Avron, Joseph E.; Osadchy, Daniel; Seiler, Ruedi (2003)."A Topological Look at the Quantum Hall Effect".Physics Today.56(8): 38–42.Bibcode:2003PhT....56h..38A.doi:10.1063/1.1611351.
- ^David J Thouless (12 March 1998).Topological Quantum Numbers in Nonrelativistic Physics.World Scientific.ISBN978-981-4498-03-6.
- ^Wen, Xiao-Gang (1992)."Theory of the edge states in fractional quantum Hall effects"(PDF).International Journal of Modern Physics C.6(10): 1711–1762.Bibcode:1992IJMPB...6.1711W.CiteSeerX10.1.1.455.2763.doi:10.1142/S0217979292000840.Archived fromthe original(PDF)on 22 May 2005.Retrieved14 June2012.
- ^abcdGirvin, Steven M.; Yang, Kun (2019-02-28).Modern Condensed Matter Physics.Cambridge University Press.ISBN978-1-108-57347-4.
- ^Thouless, D. J.; Kohmoto, M.; Nightingale, M. P.; den Nijs, M. (1982-08-09)."Quantized Hall Conductance in a Two-Dimensional Periodic Potential".Physical Review Letters.49(6): 405–408.Bibcode:1982PhRvL..49..405T.doi:10.1103/PhysRevLett.49.405.
- ^Kane, C. L.; Mele, E. J. (2005-11-23)."Quantum Spin Hall Effect in Graphene".Physical Review Letters.95(22): 226801.arXiv:cond-mat/0411737.Bibcode:2005PhRvL..95v6801K.doi:10.1103/PhysRevLett.95.226801.PMID16384250.S2CID6080059.
- ^Hasan, M. Z.; Kane, C. L. (2010-11-08)."Colloquium: Topological insulators".Reviews of Modern Physics.82(4): 3045–3067.arXiv:1002.3895.Bibcode:2010RvMP...82.3045H.doi:10.1103/RevModPhys.82.3045.S2CID16066223.
- ^Bednorz, J.G., Müller, K.A. (1986), "Possible high Tc superconductivity in the Ba−La−Cu−O system.",Z. Physik B - Condensed Matter,64(2): 189–193,doi:10.1007/BF01303701
{{citation}}
:CS1 maint: multiple names: authors list (link) - ^Quintanilla, Jorge; Hooley, Chris (June 2009)."The strong-correlations puzzle"(PDF).Physics World.22(6): 32.Bibcode:2009PhyW...22f..32Q.doi:10.1088/2058-7058/22/06/38.Archived fromthe original(PDF)on 6 September 2012.Retrieved14 June2012.
- ^Eugenie Samuel Reich (2012)."Hopes surface for exotic insulator".Nature.492(7428): 165.Bibcode:2012Natur.492..165S.doi:10.1038/492165a.PMID23235853.
- ^Dzero, V.; K. Sun; V. Galitski; P. Coleman (2010). "Topological Kondo Insulators".Physical Review Letters.104(10): 106408.arXiv:0912.3750.Bibcode:2010PhRvL.104j6408D.doi:10.1103/PhysRevLett.104.106408.PMID20366446.S2CID119270507.
- ^"Understanding Emergence".National Science Foundation.Retrieved30 March2012.
- ^Levin, Michael; Wen, Xiao-Gang (2005). "Colloquium: Photons and electrons as emergent phenomena".Reviews of Modern Physics.77(3): 871–879.arXiv:cond-mat/0407140.Bibcode:2005RvMP...77..871L.doi:10.1103/RevModPhys.77.871.S2CID117563047.
- ^abNeil W. Ashcroft; N. David Mermin (1976).Solid state physics.Saunders College.ISBN978-0-03-049346-1.
- ^Eckert, Michael (2011)."Disputed discovery: the beginnings of X-ray diffraction in crystals in 1912 and its repercussions".Acta Crystallographica A.68(1): 30–39.Bibcode:2012AcCrA..68...30E.doi:10.1107/S0108767311039985.PMID22186281.
- ^Han, Jung Hoon (2010).Solid State Physics(PDF).Sung Kyun Kwan University. Archived fromthe original(PDF)on 2013-05-20.
- ^abPerdew, John P.;Ruzsinszky, Adrienn(2010)."Fourteen Easy Lessons in Density Functional Theory"(PDF).International Journal of Quantum Chemistry.110(15): 2801–2807.doi:10.1002/qua.22829.Archived(PDF)from the original on 2022-10-09.Retrieved13 May2012.
- ^Nambu, Yoichiro (8 December 2008)."Spontaneous Symmetry Breaking in Particle Physics: a Case of Cross Fertilization".Nobelprize.org.
- ^Greiter, Martin (16 March 2005). "Is electromagnetic gauge invariance spontaneously violated in superconductors?".Annals of Physics.319(2005): 217–249.arXiv:cond-mat/0503400.Bibcode:2005AnPhy.319..217G.doi:10.1016/j.aop.2005.03.008.S2CID55104377.
- ^Leutwyler, H. (1997). "Phonons as Goldstone bosons".Helv. Phys. Acta.70(1997): 275–286.arXiv:hep-ph/9609466.Bibcode:1996hep.ph....9466L.
- ^abVojta, Matthias (2003). "Quantum phase transitions".Reports on Progress in Physics.66(12): 2069–2110.arXiv:cond-mat/0309604.Bibcode:2003RPPh...66.2069V.CiteSeerX10.1.1.305.3880.doi:10.1088/0034-4885/66/12/R01.S2CID15806867.
- ^abcdCondensed-Matter Physics, Physics Through the 1990s.National Research Council. 1986.doi:10.17226/626.ISBN978-0-309-03577-4.
- ^Malcolm F. Collins Professor of Physics McMaster University (1989-03-02).Magnetic Critical Scattering.Oxford University Press, USA.ISBN978-0-19-536440-8.
- ^Richardson, Robert C. (1988).Experimental methods in Condensed Matter Physics at Low Temperatures.Addison-Wesley.ISBN978-0-201-15002-5.
- ^abChaikin, P. M.; Lubensky, T. C. (1995).Principles of condensed matter physics.Cambridge University Press.ISBN978-0-521-43224-5.
- ^Wentao Zhang (22 August 2012).Photoemission Spectroscopy on High Temperature Superconductor: A Study of Bi2Sr2CaCu2O8 by Laser-Based Angle-Resolved Photoemission.Springer Science & Business Media.ISBN978-3-642-32472-7.
- ^Siegel, R. W. (1980). "Positron Annihilation Spectroscopy".Annual Review of Materials Science.10:393–425.Bibcode:1980AnRMS..10..393S.doi:10.1146/annurev.ms.10.080180.002141.
- ^Committee on Facilities for Condensed Matter Physics (2004)."Report of the IUPAP working group on Facilities for Condensed Matter Physics: High Magnetic Fields"(PDF).International Union of Pure and Applied Physics. Archived fromthe original(PDF)on 2014-02-22.Retrieved2016-02-07.
The magnetic field is not simply a spectroscopic tool but a thermodynamic variable which, along with temperature and pressure, controls the state, the phase transitions and the properties of materials.
- ^abCommittee to Assess the Current Status and Future Direction of High Magnetic Field Science in the United States; Board on Physics and Astronomy; Division on Engineering and Physical Sciences; National Research Council (25 November 2013).High Magnetic Field Science and Its Application in the United States: Current Status and Future Directions.National Academies Press.doi:10.17226/18355.ISBN978-0-309-28634-3.
{{cite book}}
:CS1 maint: multiple names: authors list (link) - ^Moulton, W. G.; Reyes, A. P. (2006)."Nuclear Magnetic Resonance in Solids at very high magnetic fields".In Herlach, Fritz (ed.).High Magnetic Fields.Science and Technology. World Scientific.ISBN978-981-277-488-0.
- ^Doiron-Leyraud, Nicolas; et al. (2007). "Quantum oscillations and the Fermi surface in an underdoped high-Tc superconductor".Nature.447(7144): 565–568.arXiv:0801.1281.Bibcode:2007Natur.447..565D.doi:10.1038/nature05872.PMID17538614.S2CID4397560.
- ^Buluta, Iulia; Nori, Franco (2009). "Quantum Simulators".Science.326(5949): 108–11.Bibcode:2009Sci...326..108B.doi:10.1126/science.1177838.PMID19797653.S2CID17187000.
- ^Greiner, Markus; Fölling, Simon (2008). "Condensed-matter physics: Optical lattices".Nature.453(7196): 736–738.Bibcode:2008Natur.453..736G.doi:10.1038/453736a.PMID18528388.S2CID4572899.
- ^Jaksch, D.; Zoller, P. (2005). "The cold atom Hubbard toolbox".Annals of Physics.315(1): 52–79.arXiv:cond-mat/0410614.Bibcode:2005AnPhy.315...52J.CiteSeerX10.1.1.305.9031.doi:10.1016/j.aop.2004.09.010.S2CID12352119.
- ^Glanz, James (October 10, 2001)."3 Researchers Based in U.S. Win Nobel Prize in Physics".The New York Times.Retrieved23 May2012.
- ^Coleman, Piers (2015).Introduction to Many-Body Physics.Cambridge Core.doi:10.1017/CBO9781139020916.ISBN9780521864886.Retrieved2020-04-20.
- ^"Condensed Matter".Physics Pantheon.Retrieved2023-11-30.
- ^Committee on CMMP 2010; Solid State Sciences Committee; Board on Physics and Astronomy; Division on Engineering and Physical Sciences, National Research Council (21 December 2007).Condensed-Matter and Materials Physics: The Science of the World Around Us.National Academies Press.doi:10.17226/11967.ISBN978-0-309-13409-5.
{{cite book}}
:CS1 maint: multiple names: authors list (link) CS1 maint: numeric names: authors list (link) - ^abcYeh, Nai-Chang (2008)."A Perspective of Frontiers in Modern Condensed Matter Physics"(PDF).AAPPS Bulletin.18(2).Retrieved19 June2018.
- ^Kudernac, Tibor; Ruangsupapichat, Nopporn; Parschau, Manfred; Maciá, Beatriz; Katsonis, Nathalie; Harutyunyan, Syuzanna R.; Ernst, Karl-Heinz; Feringa, Ben L. (2011-11-01)."Electrically driven directional motion of a four-wheeled molecule on a metal surface".Nature.479(7372): 208–211.Bibcode:2011Natur.479..208K.doi:10.1038/nature10587.ISSN1476-4687.PMID22071765.S2CID6175720.
Further reading
[edit]- Anderson, Philip W. (2018-03-09).Basic Notions Of Condensed Matter Physics.CRC Press.ISBN978-0-429-97374-1.
- Girvin, Steven M.; Yang, Kun (2019-02-28).Modern Condensed Matter Physics.Cambridge University Press.ISBN978-1-108-57347-4.
- Coleman, Piers (2015).Introduction to Many-Body Physics,Cambridge University Press,ISBN0-521-86488-7.
- P. M. Chaikin and T. C. Lubensky (2000).Principles of Condensed Matter Physics,Cambridge University Press; 1st edition,ISBN0-521-79450-1
- Alexander Altland and Ben Simons (2006).Condensed Matter Field Theory,Cambridge University Press,ISBN0-521-84508-4.
- Michael P. Marder (2010).Condensed Matter Physics, second edition,John Wiley and Sons,ISBN0-470-61798-5.
- Lillian Hoddeson, Ernest Braun, Jürgen Teichmann and Spencer Weart, eds. (1992).Out of the Crystal Maze: Chapters from the History of Solid State Physics,Oxford University Press,ISBN0-19-505329-X.
External links
[edit]Media related toCondensed matter physicsat Wikimedia Commons