Graphite oxide

Graphite oxide(GO), formerly calledgraphitic oxideorgraphitic acid,is a compound ofcarbon,oxygen,andhydrogenin variable ratios, obtained by treatinggraphitewith strongoxidizersandacidsfor resolving of extrametals.The maximallyoxidizedbulk product is a yellow solid with C:O ratio between 2.1 and 2.9, that retains the layer structure of graphite but with a much larger and irregular spacing.[2][3]
The bulk material spontaneously disperses inbasicsolutions or can be dispersed bysonicationin polar solvents to yield monomolecular sheets, known asgraphene oxideby analogy tographene,the single-layer form of graphite.[4]Graphene oxide sheets have been used to prepare strong paper-like materials, membranes, thin films, and composite materials. Initially, graphene oxide attracted substantial interest as a possible intermediate for the manufacture of graphene. The graphene obtained by reduction of graphene oxide still has many chemical and structural defects which is a problem for some applications but an advantage for some others.[5][6]
History and preparation
[edit]Graphite oxide was first prepared byOxfordchemistBenjamin C. Brodiein 1859 by treating graphite with a mixture ofpotassium chlorateand fumingnitric acid.[7]He reported synthesis of "paper-like foils" with 0.05 mm thickness. In 1957 Hummers and Offeman developed a safer, quicker, and more efficient process calledHummers' method,using a mixture ofsulfuric acidH2SO4,sodium nitrateNaNO3,andpotassium permanganateKMnO4,which is still widely used, often with some modifications.[2][8][9]Largest monolayer GO with highly intact carbon framework and minimal residual impurity concentrations can be synthesized in inert containers using highly pure reactants and solvents.[10]
Graphite oxides demonstrate considerable variation of properties depending on the degree of oxidation and the synthesis method.[11][12]For example, the temperature point of explosive exfoliation is generally higher for graphite oxide prepared by the Brodie method compared to Hummers graphite oxide, the difference is up to 100 degrees with the same heating rates.[13]Hydration and solvation properties of Brodie and Hummers graphite oxides are also remarkably different.[14]
Recently a mixture of H2SO4and KMnO4has been used to cut opencarbon nanotubeslengthwise, resulting in microscopic flatribbons of graphene,a few atoms wide, with the edges "capped" by oxygen atoms (=O) or hydroxyl groups (-OH).[15]
Graphite (graphene) oxide has also been prepared by using a "bottom-up" synthesis method (Tang-Lau method) in which the sole source is glucose, the process is safer, simpler, and more environmentally friendly compared to traditionally "top-down" method, in which strong oxidizers are involved. Another important advantage of the Tang-Lau method is the control of thickness, ranging from monolayer to multilayers, by adjusting growth parameters.[16]
Structure
[edit]The structure and properties of graphite oxide depend on the particular synthesis method and degree of oxidation.[11][12]It typically preserves the layer structure of the parent graphite, but the layers are buckled and the interlayer spacing is about two times larger (~0.7 nm) than that of graphite. Strictly speaking "oxide" is an incorrect but historically established name. Besidesepoxidegroups (bridging oxygen atoms), other functional groups found experimentally are:[11]carbonyl(C=O),hydroxyl(-OH),phenoland for graphite oxides prepared using sulphuric acid (e.g. Hummers method) some impurity of sulphur is often found, for example in a form of organosulfate groups.[17][18][19][20][21][22]The detailed structure is still not understood due to the strong disorder and irregular packing of the layers.
Graphene oxide layers are about 1.1 ± 0.2 nm thick.[17][18]Scanning tunneling microscopyshows the presence of local regions where oxygen atoms are arranged in a rectangular pattern withlattice constant0.27 nm × 0.41 nm.[18][23]The edges of each layer are terminated withcarboxylandcarbonylgroups.[17]X-ray photoelectron spectroscopyshows the presence of several C1speaks, their number and relative intensity depending on the particular oxidation method used. Assignment of these peaks to certain carbon functionalization types is somewhat uncertain and still under debate. For example, one interpretation goes as follows: non-oxygenated ring contexts (284.8 eV), C-O (286.2 eV), C=O (287.8 eV) and O-C=O (289.0 eV).[24]Another interpretation, usingdensity functional theorycalculation, goes as follows: C=C with defects such as functional groups and pentagons (283.6 eV), C=C (non-oxygenated ring contexts) (284.3 eV), sp3C-H in the basal plane and C=C with functional groups (285.0 eV), C=O and C=C with functional groups, C-O (286.5 eV), and O-C=O (288.3 eV).[25]
Graphite oxide is hydrophilic and easilyhydratedwhen exposed to water vapor or immersed in liquid water, resulting in a distinct increase of the inter-planar distance (up to 1.2 nm in saturated state). Additional water is also incorporated into the interlayer space due to high pressure induced effects.[26]The maximal hydration state of graphite oxide in liquid water corresponds to insertion of 2-3 water monolayers. Cooling the graphite oxide/H2O samples results in "pseudo-negative thermal expansion" and cooling below the freezing point of water results in de-insertion of one water monolayer and lattice contraction.[14]Complete removal of water from the structure seems difficult since heating at 60–80 °C results in partial decomposition and degradation of the material.

Similar to water, graphite oxide easily incorporates other polar solvents, e.g. alcohols. However, intercalation of polar solvents occurs significantly different in Brodie and Hummers graphite oxides. Brodie graphite oxide is intercalated at ambient conditions by one monolayer of alcohols and several other solvents (e.g.dimethylformamideandacetone) when liquid solvent is available in excess. Separation of graphite oxide layers is proportional to the size of alcohol molecule.[29]Cooling of Brodie graphite oxide immersed in excess of liquidmethanol,ethanol,acetone and dimethylformamide results in step-like insertion of an additional solvent monolayer and lattice expansion. The phase transition detected byX-ray diffractionanddifferential scanning calorimetry(DSC) is reversible; de-insertion of solvent monolayer is observed when sample is heated back from low temperatures.[30]An additional methanol and ethanol monolayer is reversibly inserted into the structure of Brodie graphite oxide under high pressure conditions.[29]
Hummers graphite oxide is intercalated with two methanol or ethanol monolayers at ambient temperature. The interlayer distance of Hummers graphite oxide in an excess of liquid alcohols increases gradually upon temperature decrease, reaching 19.4 and 20.6 Å at 140 K for methanol and ethanol, respectively. The gradual expansion of the Hummers graphite oxide lattice upon cooling corresponds to insertion of at least two additional solvent monolayers.[31]
Graphite oxide exfoliates and decomposes when rapidly heated at moderately high temperatures (~280–300 °C) with formation of finely dispersedamorphous carbon,somewhat similar toactivated carbon.[28]
Characterization
[edit]
XRD,FTIR,Raman,XPS,AFM,TEM,SEM/EDX,Thermogravimetric analysis[11][32][33][25][34]etc. are some common techniques used to characterize GO samples.[35]Experimental results of graphite/graphene oxide have been analyzed by calculation in detail.[36][37]Since the distribution of oxygen functionalities on GO sheets is polydisperse, fractionation methods can be used to characterize and separate GO sheets on the basis of oxidation.[11]Different synthesis methods give rise to different types of graphene oxide. Even different batches from similar oxidation methods can have differences in their properties due to variations in purification or quenching processes.[11]
Surface properties
[edit]It is also possible to modify the surface of graphene oxide to change its properties.[33][38]Graphene oxide has unique surface properties which make it a very good surfactant material stabilizing various emulsion systems.[33][11]Graphene oxide remains at the interface of the emulsions systems due to the difference in surface energy of the two phases separated by the interface.[39][33]
Relation to water
[edit]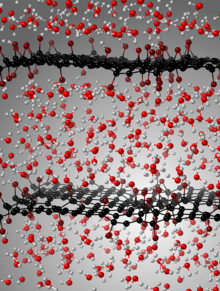
Graphite oxides absorb moisture in proportion to humidity and swell in liquid water. The amount of water absorbed by graphite oxides depends on the particular synthesis method and shows a strong temperature dependence.
Brodie graphite oxide selectively absorbs methanol from water/methanol mixtures in a certain range of methanol concentrations.[41]
Membranes prepared from graphite oxides (recently more often called "graphene oxide" membranes) are vacuum tight and impermeable to nitrogen and oxygen, but are permeable to water vapors. The membranes are also impermeable to "substances of lower molecular weight". Permeation of graphite and graphene oxide membranes by polar solvents is possible due to swelling of the graphite oxide structure.[42]The membranes in swelled state are also permeable by gases, e.g.helium.Graphene oxide sheets are chemically reactive in liquid water, leading them to acquire a small negative charge.[40]
The interlayer distance of dried graphite oxides was reported as ~6–7 Å but in liquid water it increases up to 11–13 Å at room temperature. The lattice expansion becomes stronger at lower temperatures. The inter-layer distance in dilutedNaOHreachedinfinity,resulting in dispersion of graphite oxide into single-layered graphene oxide sheets in solution. Graphite oxide can be used as a cation exchange membrane for materials such as KCl, HCl, CaCl2,MgCl2,BaCl2solutions. The membranes were permeable by large alkali ions as they are able to penetrate between graphene oxide layers.[42]
Applications
[edit]Optical nonlinearity
[edit]Nonlinear optical materials are of great importance for ultrafast photonics and optoelectronics. Recently, the giant optical nonlinearities of graphene oxide (GO) has proven useful for a number of applications.[43]For example, the optical limiting of GO is indispensable in the protection of sensitive instruments from laser-induced damage. And thesaturable absorptioncan be used for pulse compression, mode-locking and Q-switching. Also, the nonlinear refraction (Kerr effect) is crucial for applications including all-optical switching, signal regeneration, and fast optical communications.
One of the most intriguing and unique properties of GO is that its electrical and optical properties can be tuned dynamically by manipulating the content of oxygen-containing groups through either chemical or physical reduction methods. The tuning of the optical nonlinearities has been demonstrated during the laser-induced reduction process through the continuous increase of the laser irradiance, and four stages of different nonlinear activities have been discovered, which may serve as promising solid state materials for novel nonlinear functional devices.[44]And metalnanoparticlescan greatly enhance the optical nonlinearity[45]andfluorescence[46]of graphene oxide.
Graphene manufacture
[edit]Graphite oxide has attracted much interest as a possible route for the large-scale production and manipulation ofgraphene,a material with extraordinary electronic properties. Graphite oxide itself is an insulator,[47]almost asemiconductor,with differentialconductivity[11]between 1 and 5×10−3S/cm at abias voltageof 10 V.[47]However, beinghydrophilic,graphite oxide disperses readily in water, breaking up into macroscopic flakes, mostly one layer thick.Chemical reductionof these flakes would yield asuspensionof graphene flakes. It was argued that the first experimental observation ofgraphenewas reported byHanns-Peter Boehmin 1962.[48]In this early work the existence of monolayer reduced graphene oxide flakes was demonstrated. The contribution of Boehm was recently acknowledged byAndre Geim,the Nobel Prize winner for graphene research.[49]
Partial reduction can be achieved by treating the suspended graphene oxide withhydrazinehydrate at 100 °C for 24 hours,[24]by exposing graphene oxide to hydrogenplasmafor a few seconds,[47]or by exposure to a strong pulse of light, such as that of axenon flash.[50]Due to the oxidation protocol, manifold defects already present in graphene oxide hamper the effectiveness of the reduction. Thus, the graphene quality obtained after reduction is limited by the precursor quality (graphene oxide) and the efficiency of the reducing agent.[51]However, the conductivity of the graphene obtained by this route is below 10 S/cm,[50]and thecharge mobilityis between 0.1 and 10 cm2/Vs.[47][52][53]These values are much greater than the oxide's, but still a few orders of magnitude lower than those of pristine graphene.[47]Recently, the synthetic protocol for graphite oxide was optimized and almost intact graphene oxide with a preserved carbon framework was obtained. Reduction of this almost intact graphene oxide performs much better and the mobility values of charge carriers exceeds 1000 cm2/Vs for the best quality of flakes.[54]Inspection with theatomic force microscopeshows that the oxygen bonds distort the carbon layer, creating a pronounced intrinsic roughness in the oxide layers which persists after reduction. These defects also show up inRaman spectraof graphene oxide.[47]
Large amounts of graphene sheets may also be produced through thermal methods. For example, in 2006 a method was discovered that simultaneously exfoliates and reduces graphite oxide by rapid heating (>2000 °C/min) to 1050 °C. At this temperature, carbon dioxide is released as the oxygen functionalities are removed and it explosively separates the sheets as it comes out.[55]The temperature of reduction is important for the oxygen content of the final product, with higher degree of reduction for higher reduction temperatures.[56][57]
Exposing a film of graphite oxide to the laser of a LightScribe DVD has also revealed to produce quality graphene at a low cost.[58]
Graphene oxide has also been reduced to graphenein situ,using a3D printedpattern of engineeredE. colibacteria.[59]Coupling of graphene oxide with biomolecules such as peptide, proteins and enzymes enhances its biomedical applications.[60]Currently, researchers are focussed on reducing graphene oxide using non-toxic substances; tea and coffee powder, lemon extract and various plants based antioxidants are widely used.[61]
Water purification
[edit]Graphite oxides were studied fordesalinationof water usingreverse osmosisbeginning in the 1960s.[62]In 2011 additional research was released.[63]
In 2013 Lockheed Martin announced theirPerforenegraphene filter. Lockheed claims the filter reduces the energy costs of reverse osmosis desalination by 99%. Lockheed claimed that the filter was 500 times thinner than the best filter then on the market, one thousand times stronger and requires 1% of the pressure.[64]The product was not expected to be released until 2020.[65]
Another study showed that graphite oxide could be engineered to allow water to pass, but retain some larger ions.[64]Narrow capillaries allow rapid permeation by mono- or bilayer water. Multilayer laminates have a structure similar tonacre,which provides mechanical strength in water free conditions.Heliumcannot pass through the membranes in humidity free conditions, but penetrates easily when exposed to humidity, whereas water vapor passes with no resistance. Dry laminates are vacuum-tight, but immersed in water, they act as molecular sieves, blocking some solutes.[66]
A third project produced graphene sheets with subnanoscale (0.40 ± 0.24 nm) pores. The graphene was bombarded withgalliumions, which disrupt carbon bonds. Etching the result with an oxidizing solution produces a hole at each spot struck by a gallium ion. The length of time spent in the oxidizing solution determined average pore size. Pore density reached 5 trillion pores per square centimeter, while retaining structural integrity. The pores permittedcationtransport after short oxidation periods, consistent withelectrostatic repulsionfrom negatively chargedfunctional groupsat pore edges. After longer oxidation periods, sheets were permeable to salt but not larger organic molecules.[67]
In 2015 a team created a graphene oxide tea that over the course of a day removed 95% of heavy metals in a water solution.[68] A composite consisting of NiFe2O4small ferrimagnetic nanoparticles and partially reduced graphene oxide functionalized with nitrogen atoms was successfully used to remove Cr(III) ion from water. The advantage of this nanocomposite is that it can be separated from water magnetically.[69] One project layered carbon atoms in a honeycomb structure, forming a hexagon-shaped crystal that measured about 0.1 millimeters in width and length, with subnanometer holes. Later work increased the membrane size to on the order of several millimeters.[70]
Graphene attached to a polycarbonate support structure was initially effective at removing salt. However, defects formed in the graphene. Filling larger defects with nylon and small defects withhafniummetal followed by a layer of oxide restored the filtration effect.[70]
In 2016 engineers developed graphene-based films powered by the sun that can filter dirty/salty water. Bacteria were used to produce a material consisting of twonanocelluloselayers. The lower layer contains pristinecellulose,while the top layer contains cellulose and graphene oxide, which absorbs sunlight and produces heat. The system draws water from below into the material. The water diffuses into the higher layer, where it evaporates and leaves behind any contaminants. The evaporate condenses on top, where it can be captured. The film is produced by repeatedly adding a fluid coating that hardens. Bacteria produce nanocellulose fibers with interspersed graphene oxide flakes. The film is light and easily manufactured at scale.[71]
Coating
[edit]Optically transparent, multilayer films made fromgraphene oxideare impermeable under dry conditions. Exposed to water (or water vapor), they allow passage of molecules below a certain size. The films consist of millions of randomly stacked flakes, leaving nano-sizedcapillariesbetween them. Closing these nanocapillaries using chemical reduction withhydroiodic acidcreates "reduced graphene oxide" (r-GO) films that are completely impermeable to gases, liquids or strong chemicals greater than 100 nanometers thick. Glassware or copper plates covered with such a graphene "paint" can be used as containers for corrosive acids. Graphene-coated plastic films could be used in medical packaging to improve shelf life.[72][better source needed]
Related materials
[edit]Dispersedgraphene oxide flakes can also be sifted out of the dispersion (as inpaper manufacture) and pressed to make an exceedingly stronggraphene oxide paper.[16]
Graphene oxide has been used in DNA analysis applications. The large planar surface of graphene oxide allows simultaneous quenching of multiple DNA probes labeled with different dyes, providing the detection of multiple DNA targets in the same solution. Further advances in graphene oxide based DNA sensors could result in very inexpensive rapid DNA analysis.[73]Recently a group of researchers, from university of L'Aquila (Italy), discovered new wetting properties of graphene oxide thermally reduced in ultra-high vacuum up to 900 °C. They found a correlation between the surface chemical composition, the surface free energy and its polar and dispersive components, giving a rationale for the wetting properties of graphene oxide and reduced graphene oxide.[74][75]
Flexible rechargeable battery electrode
[edit]Graphene oxide has been demonstrated as a flexible free-standing battery anode material for room temperature lithium-ion[76]and sodium-ion batteries.[77][78]It is also being studied as a high surface area conducting agent in lithium-sulfur battery cathodes.[79]The functional groups on graphene oxide can serve as sites for chemical modification and immobilization of active species. This approach allows for the creation of hybrid architectures for electrode materials. Recent examples of this have been implemented in lithium-ion batteries, which are known for being rechargeable at the cost of low capacity limits. Graphene oxide-based composites functionalized with metal oxides and sulfides have been shown in recent research to induce enhanced battery performance. This has similarly been adapted into applications in supercapacitors, since the electronic properties of graphene oxide allow it to bypass some of the more prevalent restrictions of typical transition metal oxide electrodes. Research in this field is developing, with additional exploration into methods involving nitrogen doping and pH adjustment to improve capacitance.[80]Additionally, research into reduced graphene oxide sheets, which display superior electronic properties akin to pure graphene, is currently being explored. Reduced graphene oxide greatly increases the conductivity and efficiency, while sacrificing some flexibility and structural integrity.[81][82][83]
Graphene oxide lens
[edit]This sectionneeds additional citations forverification.(January 2017) |
The optical lens has been playing a critical role in almost all areas of science and technology since its invention about 3000 years ago. With the advances in micro- and nanofabrication techniques, continued miniaturization of the conventional optical lenses has always been requested for various applications such as communications, sensors, data storage and a wide range of other technology-driven and consumer-driven industries. Specifically, ever smaller sizes, as well as thinner thicknesses of micro lenses, are highly needed for subwavelength optics or nano-optics with extremely small structures, particularly for visible and near-IR applications. Also, as the distance scale for optical communications shrinks, the required feature sizes of micro lenses are rapidly pushed down.
Recently, the excellent properties of newly discovered graphene oxide provide novel solutions to overcome the challenges of current planar focusing devices. Specifically,giant refractive index modification(as large as 10^-1), which is one order of magnitude larger than the current materials, between graphene oxide (GO) and reduced graphene oxide (rGO) have been demonstrated by dynamically manipulating its oxygen content using thedirect laser writing(DLW) method. As a result, the overall lens thickness can be potentially reduced by more than ten times. Also, the linear optical absorption of GO is found to increase as the reduction of GO deepens, which results in transmission contrast between GO and rGO and therefore provides an amplitude modulation mechanism. Moreover, both the refractive index and the optical absorption are found to be dispersionless over a broad wavelength range from visible to near infrared. Finally, GO film offers flexible patterning capability by using the maskless DLW method, which reduces the manufacturing complexity and requirements.
As a result, a novel ultrathinplanar lenson a GO thin film has been realized recently using the DLW method.[84]The distinct advantage of the GO flat lens is that phase modulation and amplitude modulation can be achieved simultaneously, which are attributed to the giant refractive index modulation and the variable linear optical absorption of GO during its reduction process, respectively. Due to the enhanced wavefront shaping capability, the lens thickness is pushed down to subwavelength scale (~200 nm), which is thinner than all currentdielectric lenses(~ μm scale). The focusing intensities and the focal length can be controlled effectively by varying the laser powers and the lens sizes, respectively. By using an oil immersion highnumerical aperture(NA) objective during DLW process, 300 nm fabrication feature size on GO film has been realized, and therefore the minimum lens size has been shrunk down to 4.6 μm in diameter, which is the smallest planar micro lens and can only be realized with metasurface by FIB. Thereafter, the focal length can be reduced to as small as 0.8 μm, which would potentially increase the numerical aperture (NA) and the focusing resolution.
The full-width at half-maximum (FWHM) of 320 nm at the minimum focal spot using a 650 nm input beam has been demonstrated experimentally, which corresponding to the effective NA of 1.24 (n=1.5), the largest NA of current micro lenses. Furthermore, ultra-broadband focusing capability from 500 nm to as far as 2 μm have been realized with the same planar lens, which is still a major challenge of focusing in infrared range due to limited availability of suitable materials and fabrication technology. Most importantly, the synthesized high quality GO thin films can be flexibly integrated on various substrates and easily manufactured by using the one-step DLW method over a large area at a comparable low cost and power (~nJ/pulse), which eventually makes the GO flat lenses promising for various practical applications.
Energy conversion
[edit]Photocatalytic water splitting is an artificial photosynthesis process in which water is dissociated into hydrogen (H2) and oxygen (O2), using artificial or natural light. Methods such as photocatalytic water splitting are currently being investigated to produce hydrogen as a clean source of energy. The superior electron mobility and high surface area of graphene oxide sheets suggest it may be implemented as a catalyst that meets the requirements for this process. Specifically, graphene oxide's compositional functional groups of epoxide (-O-) and hydroxide (-OH) allow for more flexible control in the water splitting process. This flexibility can be used to tailor the band gap and band positions that are targeted in photocatalytic water splitting. Recent research experiments have demonstrated that the photocatalytic activity of graphene oxide containing a band gap within the required limits has produced effective splitting results, particularly when used with 40-50% coverage at a 2:1 hydroxide:epoxide ratio. When used in composite materials withCdS(a typical catalyst used in photocatalytic water splitting), graphene oxide nanocomposites have been shown to exhibit increasedhydrogen productionand quantum efficiency.
Hydrogen storage
[edit]Graphene oxide is also being explored for its applications in hydrogen storage. Hydrogen molecules can be stored among the oxygen-based functional groups found throughout the sheet. This hydrogen storage capability can be further manipulated by modulating the interlayer distance between sheets, as well as making changes to the pore sizes. Research in transition metal decoration on carbon sorbents to enhance hydrogen binding energy has led to experiments withtitaniumandmagnesiumanchored to hydroxyl groups, allowing for the binding of multiple hydrogen molecules.
Precision medicine
[edit]Graphene oxide has been studied for its promising uses in a wide variety ofnanomedicalapplications includingtissue engineering,cancer treatment,medical imaging,anddrug delivery.[85]Its physiochemical properties allow for a structure to regulate the behaviour ofstem cells,with the potential to assist in the intracellular delivery ofDNA,growth factors,and syntheticproteinsthat could allow for the repair and regeneration ofmuscle tissue.[86]Due to its unique behaviour in biological environments, GO has also been proposed as a novel material in earlycancer diagnosis.[87]
It has also been explored for its uses invaccinesandimmunotherapy,including as a dual-useadjuvantand carrier ofbiomedical materials.[88][89]In September 2020, researchers at the Shanghai National Engineering Research Center for Nanotechnology in China filed a patent for use of graphene oxide in arecombinant vaccineunder development againstSARS-CoV-2.[90]
Toxicity
[edit]Several typical mechanisms underlying graphene (oxide) nanomaterial's toxicity have been revealed, for instance, physical destruction, oxidative stress, DNA damage, inflammatory response, apoptosis, autophagy, and necrosis. In these mechanisms,toll-like receptors(TLR),transforming growth factor-beta(TGF-β) andtumor necrosis factor-alpha(TNF-α) dependent-pathways are involved in the signalling pathway network, and oxidative stress plays a crucial role in these pathways. Many experiments have shown that graphene (oxide)nanomaterialshave toxic side effects in many biological applications, but more in-depth study of toxicity mechanisms is needed.[91]According to the USA FDA, graphene, graphene oxide, and reduced graphene oxide elicit toxic effects both in vitro and in vivo.[92]Graphene-family nanomaterials (GFN) are not approved by the USA FDA for human consumption.
See also
[edit]References
[edit]- ^He, H.; Klinowski, J.; Forster, M.; Lerf, A. (1998). "A new structural model for graphite oxide".Chemical Physics Letters.287(1): 53.Bibcode:1998CPL...287...53H.doi:10.1016/S0009-2614(98)00144-4.
- ^abHummers, W. S.; Offeman, R. E. (1958). "Preparation of Graphitic Oxide".Journal of the American Chemical Society.80(6): 1339.doi:10.1021/ja01539a017.
- ^Sadri, Rad (2017)."Experimental study on thermo-physical and rheological properties of stable and green reduced graphene oxide nanofluids: Hydrothermal assisted technique".Journal of Dispersion Science and Technology.38(9): 1302–1310.doi:10.1080/01932691.2016.1234387.S2CID53349683.
- ^Dreyer, D. R.; Park, S.; Bielawski, C. W.; Ruoff, R. S. (2010). "The chemistry of graphene oxide".Chemical Society Reviews.39(1): 228–240.doi:10.1039/b917103g.PMID20023850.S2CID18364219.
- ^Wei, X.-D.; Mao, L.; Soler-Crespo, R. A.; Paci, J. T.; Huang, J.-X.; Nguyen, S. T.; Espinoza, H. D. (2015)."Plasticity and ductility in graphene oxide through a mechanochemically induced damage tolerance mechanism".Nature Communications.6:8029.Bibcode:2015NatCo...6.8029W.doi:10.1038/ncomms9029.PMC4560785.PMID26289729.
- ^Structural, functional and magnetic ordering modifications in graphene oxide and graphite by 100 MeV gold ion irradiation, Vacuum, Volume 182, December 2020, 109700, DOI:https://doi.org/10.1016/j.vacuum.2020.109700
- ^Brodie, B. C. (1859)."On the Atomic Weight of Graphite".Philosophical Transactions of the Royal Society of London.149:249–259.Bibcode:1859RSPT..149..249B.doi:10.1098/rstl.1859.0013.JSTOR108699.
- ^Kovtyukhova, N.I.; Ollivier, P.J.; Martin, B.J.; Mallouk, T.E.; Chizhik, S.A.; Buzaneva, E.V.; Gorchinskiy, A.D. (January 1999). "Layer-by-Layer Assembly of Ultrathin Composite Films from Micron-Size Graphite Oxide Sheets and Polycations".Chemistry of Materials.11(3): 771–778.doi:10.1021/cm981085u.
- ^Marcano, D. C.; Kosynkin, D. V.; Berlin, J. M.; Sinitskii, A.; Sun, Z.; Slesarev, A.; Alemany, L. B.; Lu, W.; Tour, J. M. (2010). "Improved Synthesis of Graphene Oxide".ACS Nano.4(8): 4806–4814.CiteSeerX10.1.1.456.3422.doi:10.1021/nn1006368.PMID20731455.
- ^Butz, Benjamin; Dolle, Christian; Halbig, Christian E.; Spiecker, Erdmann; Eigler, Siegfried (2016-12-19). "Highly Intact and Pure Oxo-Functionalized Graphene: Synthesis and Electron-Beam-Induced Reduction".Angewandte Chemie International Edition.55(51): 15771–15774.doi:10.1002/anie.201608377.ISSN1521-3773.PMID27865029.
- ^abcdefghiKumar, Harish V.; Woltornist, Steven J.; Adamson, Douglas H. (March 2016)."Fractionation and Characterization of Graphene Oxide by Oxidation Extent Through Emulsion Stabilization".Carbon.98:491–495.Bibcode:2016Carbo..98..491K.doi:10.1016/j.carbon.2015.10.083.
- ^abFeicht, Patrick; Siegel, Renée; Thurn, Herbert; Neubauer, Jens W.; Seuss, Maximilian; Szabó, Tamás; Talyzin, Alexandr V.; Halbig, Christian E.; Eigler, Siegfried (April 2017)."Systematic evaluation of different types of graphene oxide in respect to variations in their in-plane modulus"(PDF).Carbon.114:700–705.Bibcode:2017Carbo.114..700F.doi:10.1016/j.carbon.2016.12.065.ISSN0008-6223.
- ^Boehm, H. -P.; Scholz, W. (1965). "Der" Verpuffungspunkt "des Graphitoxids".Zeitschrift für Anorganische und Allgemeine Chemie.335(1–2): 74–79.doi:10.1002/zaac.19653350107.
- ^abYou, S.; Luzan, S. M.; Szabó, T. S.; Talyzin, A. V. (2013). "Effect of synthesis method on solvation and exfoliation of graphite oxide".Carbon.52:171–180.Bibcode:2013Carbo..52..171Y.doi:10.1016/j.carbon.2012.09.018.
- ^Kosynkin, D. V.; Higginbotham, A. L.; Sinitskii, A.; Lomeda, J. R.; Dimiev, A.; Price, B. K.; Tour, J. M. (2009). "Longitudinal unzipping of carbon nanotubes to form graphene nanoribbons".Nature.458(7240): 872–876.Bibcode:2009Natur.458..872K.doi:10.1038/nature07872.hdl:10044/1/4321.PMID19370030.S2CID2920478.
- ^abTang, L.; Li, X.; Ji, R.; Teng, K. S.; Tai, G.; Ye, J.; Wei, C.; Lau, S. P. (2012). "Bottom-up synthesis of large-scale graphene oxide nanosheets".Journal of Materials Chemistry.22(12): 5676.doi:10.1039/C2JM15944A.hdl:10397/15682.
- ^abcSchniepp, H. C.; Li, J. L.; McAllister, M. J.; Sai, H.; Herrera-Alonso, M.; Adamson, D. H.; Prud'Homme, R. K.; Car, R.; Saville, D. A.; Aksay, I. A. (2006). "Functionalized Single Graphene Sheets Derived from Splitting Graphite Oxide".The Journal of Physical Chemistry B.110(17): 8535–8539.CiteSeerX10.1.1.504.4994.doi:10.1021/jp060936f.PMID16640401.
- ^abcPandey, D.; Reifenberger, R.; Piner, R. (2008). "Scanning probe microscopy study of exfoliated oxidized graphene sheets".Surface Science.602(9): 1607.Bibcode:2008SurSc.602.1607P.doi:10.1016/j.susc.2008.02.025.
- ^Eigler, S.; Dotzer, C.; Hof, F.; Bauer, W.; Hirsch, A. (2013). "Sulfur Species in Graphene Oxide".Chemistry: A European Journal.19(29): 9490–6.doi:10.1002/chem.201300387.PMID23780799.
- ^Feicht, Patrick; Kunz, Daniel A.; Lerf, Anton; Breu, Josef (December 2014). "Facile and scalable one-step production of organically modified graphene oxide by a two-phase extraction".Carbon.80:229–234.Bibcode:2014Carbo..80..229F.doi:10.1016/j.carbon.2014.08.061.ISSN0008-6223.
- ^Lerf, Anton; He, Heyong; Forster, Michael; Klinowski, Jacek (June 1998). "Structure of Graphite Oxide Revisited‖".The Journal of Physical Chemistry B.102(23): 4477–4482.doi:10.1021/jp9731821.ISSN1520-6106.
- ^Szabó,†,‡, Tamás; Berkesi,§, Ottó; Forgó,‖, Péter; Josepovits,⊥, Katalin; Sanakis,✗, Yiannis; and, Dimitris Petridis,✗; Dékány*,†,○, Imre (2006-05-04). "Evolution of Surface Functional Groups in a Series of Progressively Oxidized Graphite Oxides".Chemistry of Materials.18(11): 2740–2749.doi:10.1021/cm060258.
{{cite journal}}
:CS1 maint: multiple names: authors list (link) - ^Mkhoyan, K. A.; Contryman, A. W.; Silcox, J.; Stewart, D. A.; Eda, G.; Mattevi, C.; Miller, S.; Chhowalla, M. (2009). "Atomic and Electronic Structure of Graphene-Oxide".Nano Letters.9(3): 1058–1063.Bibcode:2009NanoL...9.1058M.CiteSeerX10.1.1.455.5865.doi:10.1021/nl8034256.PMID19199476.S2CID2974943.
- ^abStankovich, S.; Piner, R. D.; Chen, X.; Wu, N.; Nguyen, S. T.; Ruoff, R. S. (2006). "Stable aqueous dispersions of graphitic nanoplatelets via the reduction of exfoliated graphite oxide in the presence of poly(sodium 4-styrenesulfonate)".Journal of Materials Chemistry.16(2): 155.doi:10.1039/b512799h.
- ^abYamada, Y.; Yasuda, H.; Murota, K.; Nakamura, M.; Sodesawa, T.; Sato, S. (2013). "Analysis of heat-treated graphite oxide by X-ray photoelectron spectroscopy".Journal of Materials Science.48(23): 8171.Bibcode:2013JMatS..48.8171Y.doi:10.1007/s10853-013-7630-0.S2CID96586004.
- ^Talyzin, A. V.; Solozhenko, V. L.; Kurakevych, O. O.; Szabó, T. S.; Dékány, I.; Kurnosov, A.; Dmitriev, V. (2008). "Colossal Pressure-Induced Lattice Expansion of Graphite Oxide in the Presence of Water".Angewandte Chemie International Edition.47(43): 8268–71.doi:10.1002/anie.200802860.PMID18814163.
- ^"Graphite oxide exfoliation by heating: exlplosion with fire".YouTube. 2011-02-03.Retrieved2013-03-18.
- ^abTalyzin, A. V.; Szabó, T. S.; DéKáNy, I.; Langenhorst, F.; Sokolov, P. S.; Solozhenko, V. L. (2009). "Nanocarbons by High-Temperature Decomposition of Graphite Oxide at Various Pressures".The Journal of Physical Chemistry C.113(26): 11279.doi:10.1021/jp9016272.
- ^abTalyzin, A. V.; Sundqvist, B.; Szabó, T. S.; DéKáNy, I.; Dmitriev, V. (2009). "Pressure-Induced Insertion of Liquid Alcohols into Graphite Oxide Structure".Journal of the American Chemical Society.131(51): 18445–18449.doi:10.1021/ja907492s.PMID19947629.
- ^You, S.; Luzan, S.; Yu, J.; Sundqvist, B.; Talyzin, A. V. (2012)."Phase Transitions in Graphite Oxide Solvates at Temperatures Near Ambient".The Journal of Physical Chemistry Letters.3(7): 812–7.doi:10.1021/jz300162u.PMID26286402.
- ^You, S.; Sundqvist, B.; Talyzin, A. V. (2013)."Enormous Lattice Expansion of Hummers Graphite Oxide in Alcohols at Low Temperatures".ACS Nano.7(2): 1395–1399.doi:10.1021/nn3051105.PMID23297717.
- ^Kazemi, E (2016-01-15). "Iron oxide functionalized graphene oxide as an efficient sorbent for dispersive micro-solid phase extraction of sulfadiazine followed by spectrophotometric and mode-mismatched thermal lens spectrometric determination".Talanta.147:561–568.doi:10.1016/j.talanta.2015.10.033.PMID26592647.
- ^abcdKumar, Harish V.; Huang, Kevin Y. -S.; Ward, Shawn P.; Adamson, Douglas H. (2017-05-01)."Altering and investigating the surfactant properties of graphene oxide".Journal of Colloid and Interface Science.493:365–370.Bibcode:2017JCIS..493..365K.doi:10.1016/j.jcis.2017.01.043.PMID28126609.
- ^Farivar, Farzaneh; Lay Yap, Pei; Karunagaran, Ramesh Udayashankar; Losic, Dusan (2021-04-27)."Thermogravimetric Analysis (TGA) of Graphene Materials: Effect of Particle Size of Graphene, Graphene Oxide and Graphite on Thermal Parameters".C.7(2): 41.doi:10.3390/c7020041.hdl:2440/140040.ISSN2311-5629.
- ^Marcano, Daniela C.; Kosynkin, Dmitry V.; Berlin, Jacob M.; Sinitskii, Alexander; Sun, Zhengzong; Slesarev, Alexander; Alemany, Lawrence B.; Lu, Wei; Tour, James M. (2010-08-24). "Improved Synthesis of Graphene Oxide".ACS Nano.4(8): 4806–4814.CiteSeerX10.1.1.456.3422.doi:10.1021/nn1006368.ISSN1936-0851.PMID20731455.
- ^Yamada, Y.; Yasuda, H.; Murota, K.; Nakamura, M.; Sodesawa, T.; Sato, S. (2013). "Analysis of heat-treated graphite oxide by X-ray photoelectron spectroscopy".Journal of Materials Science.48(23): 8171.Bibcode:2013JMatS..48.8171Y.doi:10.1007/s10853-013-7630-0.S2CID96586004.
- ^Kudin, Konstantin N.; Ozbas, Bulent; Schniepp, Hannes C.; Prud'homme, Robert K.; Aksay, Ilhan A.; Car, Roberto (2008-01-01). "Raman Spectra of Graphite Oxide and Functionalized Graphene Sheets".Nano Letters.8(1): 36–41.Bibcode:2008NanoL...8...36K.doi:10.1021/nl071822y.ISSN1530-6984.PMID18154315.
- ^Wu, Cheng-Ken; Wang, Guo-Jian; Dai, Jin-Feng (2013-05-01). "Controlled functionalization of graphene oxide through surface modification with acetone".Journal of Materials Science.48(9): 3436–3442.Bibcode:2013JMatS..48.3436W.doi:10.1007/s10853-012-7131-6.ISSN0022-2461.S2CID95458738.
- ^Kim, Jaemyung; Cote, Laura J.; Kim, Franklin; Yuan, Wa; Shull, Kenneth R.; Huang, Jiaxing (2010-06-16). "Graphene Oxide Sheets at Interfaces".Journal of the American Chemical Society.132(23): 8180–8186.doi:10.1021/ja102777p.ISSN0002-7863.PMID20527938.
- ^abMouhat, Félix; Coudert, François-Xavier; Bocquet, Marie-Laure (2020-03-26)."Structure and chemistry of graphene oxide in liquid water from first principles".Nature Communications.11(1): 1566.arXiv:1911.04987.Bibcode:2020NatCo..11.1566M.doi:10.1038/s41467-020-15381-y.ISSN2041-1723.PMC7099009.PMID32218448.
- ^You, S.; Yu, J.; Sundqvist, B.; Belyaeva, L. A.; Avramenko, N. V.; Korobov, M. V.; Talyzin, A. V. (2013)."Selective Intercalation of Graphite Oxide by Methanol in Water/Methanol Mixtures".The Journal of Physical Chemistry C.117(4): 1963.doi:10.1021/jp312756w.
- ^abH.P.Boehm, A.Clauss, U Hoffmann (1960). "Graphite oxide and its membrane properties".J. Chim. Phys. Rev. Gén. Colloïdes.58(12): 110–117.
{{cite journal}}
:CS1 maint: multiple names: authors list (link) - ^Liu, Zhibo; Wang, Yan; Zhang, Xiaoliang; Xu, Yanfei; Chen, Yongsheng; Tian, Jianguo (January 12, 2009). "Nonlinear optical properties of graphene oxide in nanosecond and picosecond regimes".Applied Physics Letters.94(2): 021902.Bibcode:2009ApPhL..94b1902L.doi:10.1063/1.3068498.
- ^Zheng, Xiaorui;Jia, Baohua;Chen, Xi; Gu, Min (May 7, 2014). "In Situ Third-Order Non-linear Responses During Laser Reduction of Graphene Oxide Thin Films Towards On-Chip Non-linear Photonic Devices".Advanced Materials.26(17): 2699–2703.Bibcode:2014AdM....26.2699Z.doi:10.1002/adma.201304681.hdl:1959.3/375725.PMID24639376.S2CID205252959.
- ^Fakhri, P (2016). "Nonlocal nonlinear optical response of graphene oxide- Au nanoparticles dispersed in different solvents".Journal of Optics.18(1): 015502.Bibcode:2016JOpt...18a5502F.doi:10.1088/2040-8978/18/1/015502.S2CID124734692.
- ^Omidvar, A (2016)."Metal-enhanced fluorescence of graphene oxide by palladium nanoparticles in the blue-green part of the spectrum".Chinese Physics B.25(11): 118102.Bibcode:2016ChPhB..25k8102O.doi:10.1088/1674-1056/25/11/118102.S2CID125102995.
- ^abcdefGómez-Navarro, C.; Weitz, R. T.; Bittner, A. M.; Scolari, M.; Mews, A.; Burghard, M.; Kern, K. (2007). "Electronic Transport Properties of Individual Chemically Reduced Graphene Oxide Sheets".Nano Letters.7(11): 3499–3503.Bibcode:2007NanoL...7.3499G.doi:10.1021/nl072090c.PMID17944526.
- ^Sprinkle, Mike (2009-12-07)."Boehm's 1961 isolation of graphene".Graphene Times.Archived fromthe originalon 2010-10-08.
- ^"Letters to the Editor".APS News.19(1). January 2010.
- ^abCote, L. J.; Cruz-Silva, R.; Huang, J. (2009). "Flash Reduction and Patterning of Graphite Oxide and Its Polymer Composite".Journal of the American Chemical Society.131(31): 11027–11032.CiteSeerX10.1.1.621.9038.doi:10.1021/ja902348k.PMID19601624.
- ^Eigler, S.; Grimm, S.; Enzelberger-Heim, M.; Müller, P.; Hirsch, A. (2013)."Graphene oxide: Efficiency of reducing agents".Chemical Communications.49(67): 7391–7393.doi:10.1039/C3CC43612H.PMID23860424.
- ^GóMez-Navarro, C.; Weitz, R. T.; Bittner, A. M.; Scolari, M.; Mews, A.; Burghard, M.; Kern, K. (2009)."Electronic Transport Properties of Individual Chemically Reduced Graphene Oxide Sheets".Nano Letters.9(5): 2206.Bibcode:2009NanoL...9.2206G.doi:10.1021/nl901209z.
- ^Eda, G.; Ball, J.; Mattevi, C.; Acik, M.; Artiglia, L.; Granozzi, G.; Chabal, Y.; Anthopoulos, T. D.; Chhowalla, M. (2011). "Partially oxidized graphene as a precursor to graphene".Journal of Materials Chemistry.21(30): 11217.doi:10.1039/C1JM11266J.S2CID15486130.
- ^Eigler, S.; Enzelberger-Heim, M.; Grimm, S.; Hofmann, P.; Kroener, W.; Geworski, A.; Dotzer, C.; Röckert, M.; Xiao, J.; Papp, C.; Lytken, O.; Steinrück, H. P.; Müller, P.; Hirsch, A. (2013). "Wet Chemical Synthesis of Graphene".Advanced Materials.25(26): 3583–3587.Bibcode:2013AdM....25.3583E.doi:10.1002/adma.201300155.PMID23703794.S2CID26172029.
- ^Schniepp, H. C.; Li, J.-L.; McAllister, M. J.; Sai, H.; Herrera-Alonso, M.; Adamson, D. H.; Aksay, I. A. (2006). "Functionalized single graphene sheets derived from splitting graphite oxide".The Journal of Physical Chemistry B.110(17): 8535–9.CiteSeerX10.1.1.504.4994.doi:10.1021/jp060936f.PMID16640401.
- ^Lavin-Lopez, M.P.; Paton-Carrero, A.; Sanchez-Silva, L.; Valverde, J.L.; Romero, A. (December 2017)."Influence of the reduction strategy in the synthesis of reduced graphene oxide".Advanced Powder Technology.28(12): 3195–3203.doi:10.1016/j.apt.2017.09.032.hdl:10578/18152.
- ^Sengupta, Iman; Chakraborty, Samarshi; Talukdar, Monikangkana; Pal, Surjya K.; Chakraborty, Sudipto (2018-12-14)."Thermal reduction of graphene oxide: How temperature influences purity".Journal of Materials Research.33(23): 4113–4122.Bibcode:2018JMatR..33.4113S.doi:10.1557/jmr.2018.338.ISSN0884-2914.S2CID139182770.
- ^El-Kady, M. F.; Strong, V.; Dubin, S.; Kaner, R. B. (2012). "Laser Scribing of High-Performance and Flexible Graphene-Based Electrochemical Capacitors".Science.335(6074): 1326–1330.Bibcode:2012Sci...335.1326E.doi:10.1126/science.1216744.PMID22422977.S2CID18958488.
- ^Lehner, Benjamin A. E.; Schmieden, Dominik T.; Meyer, Anne S. (22 February 2017)."A Straightforward Approach for 3D Bacterial Printing".ACS Synth. Biol.6(7): 1124–1130.doi:10.1021/acssynbio.6b00395.PMC5525104.PMID28225616.
- ^Joshi, Shubhi; Chadha, Jatin; Harjai, Kusum; Verma, Gaurav; Saini, Avneet (March 2024)."Synthetic peptide (DP1) functionalized graphene oxide: A biocompatible nanoformulation with broad-spectrum antibacterial and antibiofilm activity".FlatChem.44:100626.doi:10.1016/j.flatc.2024.100626.
- ^Khan, Junaid; Jaafar, Mariatti (November 2021). "Reduction efficiencies of natural substances for reduced graphene oxide synthesis".Journal of Materials Science.56(33): 18477–18492.Bibcode:2021JMatS..5618477K.doi:10.1007/s10853-021-06492-y.S2CID237496714.
- ^E.S.Bober (1970). "Final report on reverse osmosis membranes containing graphitic oxide".U.S. Dept. of the Interior:116 pages.
- ^Gao, W.; Majumder, M.; Alemany, L. B.; Narayanan, T. N.; Ibarra, M. A.; Pradhan, B. K.; Ajayan, P. M. (2011). "Engineered Graphite Oxide Materials for Application in Water Purification".ACS Applied Materials & Interfaces.3(6): 1821–6.doi:10.1021/am200300u.PMID21568266.
- ^ab"Can Graphene Oxide Filters Unlock Our Most Abundant Water Source?".Singularity Hub. 2014-03-11.Retrieved2014-03-13.
Joshi, R. K.; Carbone, P.; Wang, F. C.; Kravets, V. G.; Su, Y.; Grigorieva, I. V.; Wu, H. A.; Geim, A. K.; Nair, R. R. (2014). "Precise and Ultrafast Molecular Sieving Through Graphene Oxide Membranes".Science.343(6172): 752–4.arXiv:1401.3134.Bibcode:2014Sci...343..752J.doi:10.1126/science.1245711.PMID24531966.S2CID13154836. - ^Alexander, David (February 20, 2015)."Lockheed testing nanotech filters for U.S. oil industry wastewater".Reuters.RetrievedApril 3,2015.
- ^Joshi, R. K.; Carbone, P.; Wang, F. C.; Kravets, V. G.; Su, Y.; Grigorieva, I. V.; Wu, H. A.; Geim, A. K.; Nair, R. R. (2014)."New multilayer graphene structure allows 'ultraprecise,' 'ultrafast' water filtering".Science.343(6172): 752–754.arXiv:1401.3134.Bibcode:2014Sci...343..752J.doi:10.1126/science.1245711.PMID24531966.S2CID13154836.Retrieved2014-03-13.
- ^"Selective nanopores in graphene dramatically improve desalination and purification".KurzweilAI.Retrieved2014-04-05.
O'Hern, S. C.; Boutilier, M. S. H.; Idrobo, J. C.; Song, Y.; Kong, J.; Laoui, T.; Atieh, M.; Karnik, R. (2014)."Selective Ionic Transport through Tunable Subnanometer Pores in Single-Layer Graphene Membranes"(PDF).Nano Letters.14(3): 1234–41.Bibcode:2014NanoL..14.1234O.doi:10.1021/nl404118f.hdl:1721.1/99472.PMID24490698.S2CID6268833. - ^PÚBLICO (15 July 2015)."Chá de grafeno extrai metais pesados da água".PÚBLICO.
- ^Ortiz- Quiñonez, Jose Luis; Cancino- Gordillo, Francisco; Pal, Umapada (2023)."Removal of Cr (III) Ions from Water Using Magnetically Separable Graphene-Oxide-Decorated Nickel Ferrite Nanoparticles".ACS Applied Nanomaterials.6(19): 18491.doi:10.1021/acsanm.3c03618.
- ^abMajcher, Kristin (June 18, 2015)."Graphene Desalination Update".Technology Review.Retrieved2015-09-26.
- ^Jeffrey, Colin (July 27, 2016)."Graphene-based sheets make dirty water drinkable simply and cheaply".newatlas.com.Retrieved2017-04-30.
- ^"A new impermeable form of graphene oxide could be the ultimate protective coating".Kurzweil.September 19, 2014.RetrievedOctober 4,2014.
- ^He, S.; Song, B.; Li, D.; Zhu, C.; Qi, W.; Wen, Y.; Wang, L.; Song, S.; Fang, H.; Fan, C. (2010)."A Graphene Nanoprobe for Rapid, Sensitive, and Multicolor Fluorescent DNA Analysis".Advanced Functional Materials.20(3): 453.doi:10.1002/adfm.200901639.S2CID136840660.
- ^Francesco Perrozzi; Salvatore Croce; Emanuele Treossi; Vincenzo Palermo; Sandro Santucci; Giulia Fioravanti; Luca Ottaviano (2014). "Reduction dependent wetting properties of graphene oxide".Carbon.77:473–480.Bibcode:2014Carbo..77..473P.doi:10.1016/j.carbon.2014.05.052.
- ^Francesco Perrozzi; Stefano Prezioso; Maurizio Donarelli; Federico Bisti; Patrizia De Marco; Sandro Santucci; Michele Nardone; Emanuele Treossi; Vincenzo Palermo; Luca Ottaviano (2013). "Use of Optical Contrast To Estimate the Degree of Reduction of Graphene Oxide".The Journal of Physical Chemistry C.117(1): 620–625.doi:10.1021/jp3069738.
- ^Coxworth, Ben (August 27, 2012)."Graphene paper anodes pave way for faster charging Li-ion batteries".www.gizmag.com.Retrieved2015-09-26.
- ^David, Lamuel; Singh, Gurpreet (2014-12-11). "Reduced Graphene Oxide Paper Electrode: Opposing Effect of Thermal Annealing on Li and Na Cyclability".The Journal of Physical Chemistry C.118(49): 28401–28408.doi:10.1021/jp5080847.ISSN1932-7447.
- ^"Research aims to improve rechargeable batteries by focusing on graphene oxide paper".
- ^"Flexible paper electrodes with ultra-high loading for lithium-sulfur batteries".October 20, 2014.
- ^Li, Fen (September 2015)."Graphene oxide: A promising nanomaterial for energy and environmental applications".Nano Energy.16:488–515.Bibcode:2015NEne...16..488L.doi:10.1016/j.nanoen.2015.07.014.
- ^De La Fuente, Jesus (September 2018)."Reduced Graphene Oxide - What Is It? How Is It Created?".www.graphenea.com.Retrieved2018-11-16.
- ^Jung, Inhwa (November 1, 2008). "Tunable Electrical Conductivity of Individual Graphene Oxide Sheets Reduced at" Low "Temperatures".Nano Letters.8(12): 4283–4287.Bibcode:2008NanoL...8.4283J.doi:10.1021/nl8019938.PMID19367929.
- ^Pei, Songfeng (December 2010). "Direct reduction of graphene oxide films into highly conductive and flexible graphene films by hydrohalic acids".Carbon.48(15): 4466–4474.Bibcode:2010Carbo..48.4466P.doi:10.1016/j.carbon.2010.08.006.
- ^"Highly efficient and ultra-broadband graphene oxide ultrathin lenses with three-dimensional subwavelength focusing".Xiaorui Zheng.September 22, 2015.RetrievedAugust 20,2015.
- ^An, Seong Soo; Wu, Si-Ying; Hulme, John (2015-08-26)."Current applications of graphene oxide in nanomedicine".International Journal of Nanomedicine.10 Spec Iss (Spec Iss): 9–24.doi:10.2147/IJN.S88285.ISSN1178-2013.PMC4554423.PMID26345988.
- ^Maleki, Masomeh; Zarezadeh, Reza; Nouri, Mohammad; Sadigh, Aydin Raei; Pouremamali, Farhad; Asemi, Zatollah; Kafil, Hossein Samadi; Alemi, Forough; Yousefi, Bahman (2020-12-31)."Graphene Oxide: A Promising Material for Regenerative Medicine and Tissue Engineering".Biomolecular Concepts.11(1): 182–200.doi:10.1515/bmc-2020-0017.ISSN1868-503X.PMID34233430.S2CID231628779.
- ^Di Santo, Riccardo; Digiacomo, Luca; Quagliarini, Erica; Capriotti, Anna Laura; Laganà, Aldo; Zenezini Chiozzi, Riccardo; Caputo, Damiano; Cascone, Chiara; Coppola, Roberto; Pozzi, Daniela; Caracciolo, Giulio (2020-05-25)."Personalized Graphene Oxide-Protein Corona in the Human Plasma of Pancreatic Cancer Patients".Frontiers in Bioengineering and Biotechnology.8:491.doi:10.3389/fbioe.2020.00491.ISSN2296-4185.PMC7261887.PMID32523944.
- ^Xu, Ligeng; Xiang, Jian; Liu, Ye; Xu, Jun; Luo, Yinchan; Feng, Liangzhu; Liu, Zhuang; Peng, Rui (2016)."Functionalized graphene oxide serves as a novel vaccine nano-adjuvant for robust stimulation of cellular immunity".Nanoscale.8(6): 3785–3795.Bibcode:2016Nanos...8.3785X.doi:10.1039/C5NR09208F.ISSN2040-3364.PMID26814441.
- ^Cao, Wanjun; He, Lin; Cao, Weidong; Huang, Xiaobing; Jia, Kun; Dai, Jingying (2020-07-11)."Recent progress of graphene oxide as a potential vaccine carrier and adjuvant".Acta Biomaterialia.112:14–28.doi:10.1016/j.actbio.2020.06.009.PMID32531395.S2CID219621172.
- ^"CN112220919 Nano coronavirus recombinant vaccine taking graphene oxide as carrier".World Intellectual Property Organization.2021-01-15.Archivedfrom the original on 2022-05-09.Retrieved2022-05-09.
- ^Ou, Lingling; Song, Bin; Liang, Huimin; Liu, Jia; Feng, Xiaoli; Deng, Bin; Sun, Ting; Shao, Longquan (2016-10-31)."Toxicity of graphene-family nanoparticles: a general review of the origins and mechanisms".Particle and Fibre Toxicology.13(1): 57.doi:10.1186/s12989-016-0168-y.ISSN1743-8977.PMC5088662.PMID27799056.
This article incorporates text available under theCC BY 4.0license.
- ^"Scientific Publications by FDA Staff".www.accessdata.fda.gov.Retrieved2021-07-07.