Outer space

Outer space(or simplyspace) is the expanse that exists beyondEarth's atmosphereand betweencelestial bodies.[1]It contains ultra-lowlevels of particle densities,constituting anear-perfect vacuum[2]of predominantlyhydrogenandheliumplasma,permeated byelectromagnetic radiation,cosmic rays,neutrinos,magnetic fieldsanddust.The baselinetemperatureof outer space, as set by thebackground radiationfrom theBig Bang,is 2.7 kelvins (−270 °C; −455 °F).[3]
Theplasma between galaxiesis thought to account for about half of thebaryonic (ordinary) matterin the universe, having anumber densityof less than onehydrogen atomper cubic metre and akinetic temperatureof millions ofkelvins.[4]Local concentrations of matter have condensed intostarsandgalaxies.Intergalactic space takes up most of the volume of theuniverse,but even galaxies andstar systemsconsist almost entirely of empty space. Most of the remainingmass-energyin theobservable universeis made up of an unknown form, dubbeddark matteranddark energy.[5][6][7][8]
Outer space does not begin at a definite altitude above Earth's surface. TheKármán line,an altitude of 100 km (62 mi) abovesea level,[9][10]is conventionally used as the start of outer space in space treaties and for aerospace records keeping. Certain portions of the upperstratosphereand themesosphereare sometimes referred to as "near space". The framework for internationalspace lawwas established by theOuter Space Treaty,which entered into force on 10 October 1967. This treaty precludes any claims ofnational sovereigntyand permits all states to freelyexplore outer space.Despite the drafting ofUN resolutionsfor the peaceful uses of outer space,anti-satellite weaponshave been tested inEarth orbit.
The concept that the space between the Earth and the Moon must be a vacuum was first proposed in the 17th century after scientists discovered thatair pressuredecreased with altitude. The immense scale of outer space was grasped in the 20th century when the distance to theAndromeda galaxywas first measured. Humans began the physical exploration of space later in the same century with the advent of high-altitudeballoon flights.This was followed by crewedrocket flightsand, then, crewed Earth orbit, first achieved byYuri Gagarinof theSoviet Unionin 1961. The economic cost of putting objects, including humans, into space is very high, limiting humanspaceflighttolow Earth orbitand theMoon.On the other hand,uncrewed spacecrafthave reached all of the knownplanetsin theSolar System.Outer space represents a challenging environment forhuman explorationbecause of the hazards ofvacuumandradiation.Microgravityhas a negative effect on humanphysiologythat causes bothmuscle atrophyandbone loss.
Terminology
The use of the short versionspace,as meaning 'the region beyond Earth's sky', predates the use of full term "outer space", with the earliest recorded use of the latter in an epic poem byJohn MiltoncalledParadise Lost,published in 1667.[11][12]
The termoutward spaceexisted in a poem from 1842 by the English poet LadyEmmeline Stuart-Wortleycalled "The Maiden of Moscow",[13]but in astronomy the termouter spacefound its application for the first time in 1845 byAlexander von Humboldt.[14]The term was eventually popularized through the writings ofH. G. Wellsafter 1901.[15]Theodore von Kármánused the term offree spaceto name the space of altitudes above Earth where spacecrafts reach conditions sufficiently free from atmospheric drag, differentiating it fromairspace,identifying a legal space above territories free from thesovereignjurisdiction of countries.[16]
"Spaceborne"denotes existing in outer space, especially if carried by a spacecraft;[17][18]similarly, "space-based"means based in outer space or on a planet or moon.[19]
Formation and state

The size of the whole universe is unknown, and it might be infinite in extent.[20]According to the Big Bang theory, the very early universe was an extremely hot and dense state about13.8 billion years ago[21]which rapidlyexpanded.About 380,000 years later the universe had cooled sufficiently to allow protons and electrons to combine and form hydrogen—the so-calledrecombination epoch.When this happened, matter and energy became decoupled, allowing photons to travel freely through the continually expanding space.[22]Matter that remained following the initial expansion has since undergone gravitational collapse to create stars, galaxies and other astronomical objects, leaving behind a deepvacuumthat forms what is now called outer space.[23]As light has a finite velocity, this theory constrains the size of the directly observable universe.[22]
The present dayshape of the universehas been determined from measurements of thecosmic microwave backgroundusing satellites like theWilkinson Microwave Anisotropy Probe.These observations indicate that thespatial geometryof the observable universe is "flat",meaning that photons on parallel paths at one point remain parallel as they travel through space to the limit of the observable universe, except for local gravity.[24]The flat universe, combined with the measured mass density of the universe and the acceleratingexpansion of the universe,indicates that space has a non-zerovacuum energy,which is calleddark energy.[25]
Estimates put the averageenergy densityof the present day universe at the equivalent of 5.9 protons per cubic meter, including dark energy, dark matter, and baryonic matter (ordinary matter composed of atoms). The atoms account for only 4.6% of the total energy density, or a density of one proton per four cubic meters.[26]The density of the universe is clearly not uniform; it ranges from relatively high density in galaxies—including very high density in structures within galaxies, such as planets, stars, andblack holes—to conditions in vastvoidsthat have much lower density, at least in terms of visible matter.[27]Unlike matter and dark matter, dark energy seems not to be concentrated in galaxies: although dark energy may account for a majority of the mass-energy in the universe, dark energy's influence is 5orders of magnitudesmaller than the influence of gravity from matter and dark matter within the Milky Way.[28]
Environment

Outer space is the closest known approximation to aperfect vacuum.It has effectively nofriction,allowing stars,planets,andmoonsto move freely along their idealorbits,following theinitial formationstage. The deep vacuum ofintergalactic spaceis not devoid ofmatter,as it contains a fewhydrogen atomsper cubic meter.[30]By comparison, the air humans breathe contains about 1025molecules per cubic meter.[31][32]The low density of matter in outer space means that electromagnetic radiation can travel great distances without being scattered: themean free pathof aphotonin intergalactic space is about 1023km, or 10 billion light years.[33]In spite of this,extinction,which is theabsorptionandscatteringof photons by dust and gas, is an important factor in galactic and intergalacticastronomy.[34]
Stars, planets, and moons retain theiratmospheresby gravitational attraction. Atmospheres have no clearly delineated upper boundary: the density of atmospheric gas gradually decreases with distance from the object until it becomes indistinguishable from outer space.[35]The Earth's atmosphericpressuredrops to about0.032Paat 100 kilometres (62 miles) of altitude,[36]compared to 100,000 Pa for theInternational Union of Pure and Applied Chemistry(IUPAC) definition ofstandard pressure.Above this altitude, isotropic gas pressure rapidly becomes insignificant when compared toradiation pressurefrom theSunand thedynamic pressureof thesolar wind.Thethermospherein this range has large gradients of pressure, temperature and composition, and varies greatly due tospace weather.[37]
The temperature of outer space is measured in terms of thekineticactivity of the gas,[38]as it is on Earth. The radiation of outer space has a different temperature than the kinetic temperature of the gas, meaning that the gas and radiation are not inthermodynamic equilibrium.[39][40]All of the observable universe is filled with photons that were created during the Big Bang, which is known as thecosmic microwave background radiation(CMB). (There is quite likely a correspondingly large number ofneutrinoscalled thecosmic neutrino background.[41]) The currentblack bodytemperature of the background radiation is about 2.7 K (−270 °C; −455 °F).[42]The gas temperatures in outer space can vary widely. For example, the temperature in theBoomerang Nebulais 1 K (−272 °C; −458 °F),[43]while thesolar coronareaches temperatures over 1,200,000–2,600,000 K (1,199,727–2,599,727 °C; 2,159,540–4,679,540 °F).[44]
Magnetic fields have been detected in the space around just about every class of celestial object. Star formation in spiral galaxies can generate small-scaledynamos,creating turbulent magnetic field strengths of around 5–10 μG.TheDavis–Greenstein effectcauses elongateddust grainsto align themselves with a galaxy's magnetic field, resulting in weak opticalpolarization.This has been used to show ordered magnetic fields that exist in several nearby galaxies.Magneto-hydrodynamicprocesses inactiveelliptical galaxiesproduce their characteristicjetsandradio lobes.Non-thermalradio sourceshave been detected even among the most distanthigh-zsources, indicating the presence of magnetic fields.[45]
Outside a protective atmosphere and magnetic field, there are few obstacles to the passage through space of energeticsubatomic particlesknown as cosmic rays. These particles have energies ranging from about 106eVup to an extreme 1020eV ofultra-high-energy cosmic rays.[46]The peak flux of cosmic rays occurs at energies of about 109eV, with approximately 87% protons, 12% helium nuclei and 1% heavier nuclei. In the high energy range, the flux ofelectronsis only about 1% of that of protons.[47]Cosmic rays can damage electronic components and pose ahealth threatto space travelers.[48]According to astronauts, likeDon Pettit,space has a burned/metallic odor that clings to their suits and equipment, similar to the scent of anarc weldingtorch.[49][50]
Human access
Effect on biology and human bodies

Despite the harsh environment, several life forms have been found that can withstand extreme space conditions for extended periods. Species of lichen carried on the ESABIOPANfacility survived exposure for ten days in 2007.[51]Seeds ofArabidopsis thalianaandNicotiana tabacumgerminated after being exposed to space for 1.5 years.[52]A strain ofBacillus subtilishas survived 559 days when exposed to low Earth orbit or a simulated Martian environment.[53]Thelithopanspermiahypothesis suggests that rocks ejected into outer space from life-harboring planets may successfully transport life forms to another habitable world. A conjecture is that just such a scenario occurred early in the history of the Solar System, with potentiallymicroorganism-bearing rocks being exchanged between Venus, Earth, and Mars.[54]
Vacuum
The lack of pressure in space is the most immediate dangerous characteristic of space to humans. Pressure decreases above Earth, reaching a level at an altitude of around 19.14 km (11.89 mi) that matches thevapor pressure of waterat thetemperature of the human body.This pressure level is called theArmstrong line,named after American physicianHarry G. Armstrong.[55]At or above the Armstrong line, fluids in the throat and lungs boil away. More specifically, exposed bodily liquids such as saliva, tears, and liquids in the lungs boil away. Hence, at this altitude, human survival requires a pressure suit, or a pressurized capsule.[56]
Out in space, sudden exposure of an unprotected human to very lowpressure,such as during a rapid decompression, can causepulmonary barotrauma—a rupture of the lungs, due to the large pressure differential between inside and outside the chest.[57]Even if the subject's airway is fully open, the flow of air through the windpipe may be too slow to prevent the rupture.[58]Rapid decompression can rupture eardrums and sinuses, bruising and blood seep can occur in soft tissues, and shock can cause an increase in oxygen consumption that leads tohypoxia.[59]
As a consequence of rapid decompression,oxygendissolved in the blood empties into the lungs to try to equalize thepartial pressuregradient. Once the deoxygenated blood arrives at the brain, humans lose consciousness after a few seconds and die of hypoxia within minutes.[60]Blood and other body fluids boil when the pressure drops below 6.3 kilopascals (1 psi), and this condition is calledebullism.[61]The steam may bloat the body to twice its normal size and slow circulation, but tissues are elastic and porous enough to prevent rupture. Ebullism is slowed by the pressure containment of blood vessels, so some blood remains liquid.[62][63]
Swelling and ebullism can be reduced by containment in apressure suit.The Crew Altitude Protection Suit (CAPS), a fitted elastic garment designed in the 1960s for astronauts, prevents ebullism at pressures as low as 2 kilopascals (0 psi).[64]Supplemental oxygen is needed at 8 km (5 mi) to provide enough oxygen for breathing and to prevent water loss, while above 20 km (12 mi) pressure suits are essential to prevent ebullism.[65]Most space suits use around 30–39 kilopascals (4–6 psi) of pure oxygen, about the same as the partial pressure of oxygen at the Earth's surface. This pressure is high enough to prevent ebullism, but evaporation of nitrogen dissolved in the blood could still causedecompression sicknessandgas embolismsif not managed.[66]
Weightlessness and radiation
Humans evolvedfor life in Earthgravity,and exposure to weightlessness has been shown to have deleterious effects on human health. Initially, more than 50% of astronauts experiencespace motion sickness.This can cause nausea and vomiting,vertigo,headaches,lethargy,and overall malaise. The duration of space sickness varies, but it typically lasts for 1–3 days, after which the body adjusts to the new environment. Longer-term exposure to weightlessness results inmuscle atrophyand deterioration of the skeleton, orspaceflight osteopenia.These effects can be minimized through a regimen of exercise.[67]Other effects include fluid redistribution, slowing of thecardiovascular system,decreased production ofred blood cells,balance disorders, and a weakening of theimmune system.Lesser symptoms include loss of body mass, nasal congestion, sleep disturbance, and puffiness of the face.[68]
During long-duration space travel, radiation can pose anacute health hazard.Exposure to high-energy, ionizingcosmic rayscan result in fatigue, nausea, vomiting, as well as damage to the immune system and changes to thewhite blood cellcount. Over longer durations, symptoms include an increased risk of cancer, plus damage to the eyes,nervous system,lungs and thegastrointestinal tract.[69]On a round-tripMarsmission lasting three years, a large fraction of the cells in an astronaut's body would be traversed and potentially damaged by high energy nuclei.[70]The energy of such particles is significantly diminished by the shielding provided by the walls of a spacecraft and can be further diminished by water containers and other barriers. The impact of the cosmic rays upon the shielding produces additional radiation that can affect the crew. Further research is needed to assess the radiation hazards and determine suitable countermeasures.[71]
Boundary

The transition between Earth's atmosphere and outer space lacks a well-defined physical boundary, with the air pressure steadily decreasing with altitude until it mixes with the solar wind. Various definitions for a practical boundary have been proposed, ranging from 30 km (19 mi) out to 1,600,000 km (990,000 mi).[16]
High-altitudeaircraft,such ashigh-altitude balloonshave reached altitudes above Earth of up to 50 km.[72]Up until 2021, the United States designated people who travel above an altitude of 50 mi (80 km) as astronauts.[73]Astronaut wingsare now only awarded to spacecraft crew members that "demonstrated activities during flight that were essential to public safety, or contributed to human space flight safety."[74]
In 2009, scientists used a Supra-Thermal Ion Imager to measure the direction and speed of ions in the atmosphere. They discovered that 118 km (73.3 mi) above Earth was the midpoint for charged particles transitioning from the gentle winds of the Earth's atmosphere to the more extreme flows of outer space, which can reach velocities well over 268 m/s (880 ft/s).[75][76]
Spacecraft have entered into a highly ellipticalorbitwith a perigee as low as 80 to 90 km (50 to 56 mi), surviving for multiple orbits.[77]At an altitude of 120 km (75 mi),[77]descending spacecraft such asNASA'sSpace Shuttlebeginatmospheric entry(termed the Entry Interface), whenatmospheric dragbecomes noticeable, thus beginning the process of switching from steering with thrusters to maneuvering with aerodynamic control surfaces.[78]
TheKármán line,established by theFédération Aéronautique Internationale,and used internationally by theUnited Nations,[16]is set at an altitude of 100 km (62 mi) as a working definition for the boundary between aeronautics and astronautics. This line is named afterTheodore von Kármán,who argued for an altitude where a vehicle would have to travel faster thanorbital velocityto derive sufficientaerodynamic liftfrom the atmosphere to support itself,[9][10]which he calculated to be at an altitude of about 83.8 km (52.1 mi),[72]distinguishing space below as the space ofaerodynamicsand airspace, and above as the space ofastronauticsandfree space.[16]
There is no internationally recognized legal altitude limit on nationalairspace,although the Kármán line is the most frequently used for this purpose. Objections have been made to setting this limit too high, as it could inhibit space activities due to concerns about airspace violations.[77]It has been argued for setting no specified singular altitude in international law, instead applying different limits depending on the case, in particular based on the craft and its purpose. Spacecraft have flown over foreign countries as low as 30 km (19 mi), as in the example of the Space Shuttle.[72]
Legal status

TheOuter Space Treatyprovides the basic framework for international space law. It covers the legal use of outer space by nation states, and includes in its definition ofouter space,the Moon, and other celestial bodies. The treaty states that outer space is free for all nation states to explore and is not subject to claims of national sovereignty, calling outer space the "province of all mankind". This status as acommon heritage of mankindhas been used, though not without opposition, to enforce the right to access and shared use of outer space for all nations equally, particularly non-spacefaring nations.[79]It prohibits the deployment ofnuclear weaponsin outer space. The treaty was passed by theUnited Nations General Assemblyin 1963 and signed in 1967 by the Union of Soviet Socialist Republics (USSR), the United States of America (USA), and the United Kingdom (UK). As of 2017, 105 state parties have either ratified or acceded to the treaty. An additional 25 states signed the treaty, without ratifying it.[80][81]
Since 1958, outer space has been the subject of multiple United Nations resolutions. Of these, more than 50 have been concerning the international co-operation in the peaceful uses of outer space and preventing an arms race in space.[82]Four additionalspace lawtreaties have been negotiated and drafted by the UN'sCommittee on the Peaceful Uses of Outer Space.Still, there remains no legal prohibition against deploying conventional weapons in space, andanti-satellite weaponshave been successfully tested by the USA, USSR, China,[83]and in 2019, India.[84]The 1979Moon Treatyturned the jurisdiction of all heavenly bodies (including the orbits around such bodies) over to the international community. The treaty has not been ratified by any nation that currently practices human spaceflight.[85]
In 1976, eight equatorial states (Ecuador,Colombia,Brazil,The Republic of the Congo,Zaire,Uganda,Kenya,andIndonesia) met inBogotá,Colombia: with their "Declaration of the First Meeting of Equatorial Countries", or theBogotá Declaration,they claimed control of the segment of the geosynchronous orbital path corresponding to each country.[86]These claims are not internationally accepted.[87]
An increasing issue of international space law and regulation has been the dangers of the growing number ofspace debris.[88]
Earth orbit
A spacecraft enters orbit when itscentripetalacceleration due to gravity is less than or equal to thecentrifugalacceleration due to the horizontal component of its velocity. For alow Earth orbit,this velocity is about 7,800 m/s (28,100 km/h; 17,400 mph);[89]by contrast, the fastest piloted airplane speed ever achieved (excluding speeds achieved by deorbiting spacecraft) was 2,200 m/s (7,900 km/h; 4,900 mph) in 1967 by theNorth American X-15.[90]
To achieve an orbit, aspacecraftmust travel faster than asub-orbital spaceflightalong an arcingtrajectory.The energy required to reach Earth orbital velocity at an altitude of 600 km (370 mi) is about 36MJ/kg, which is six times the energy needed merely to climb to the corresponding altitude.[91]Theescape velocityrequired to pull free of Earth's gravitational field altogether and move into interplanetary space is about 11,200 m/s (40,300 km/h; 25,100 mph).[92]
Orbiting spacecraft with aperigeebelow about 2,000 km (1,200 mi) are subject to drag from the Earth's atmosphere,[93]which decreases the orbital altitude. The rate of orbital decay depends on the satellite's cross-sectional area and mass, as well as variations in the air density of the upper atmosphere. At altitudes above 800 km (500 mi), orbital lifetime is measured in centuries.[94]Below about 300 km (190 mi), decay becomes more rapid with lifetimes measured in days. Once a satellite descends to 180 km (110 mi), it has only hours before it vaporizes in the atmosphere.[95]
Regions
Regions near the Earth
Space in proximity to the Earth is physically similar to the remainder of interplanetary space, but is home to a multitude of Earth–orbiting satellites and has been subject to extensive studies. For identification purposes, this volume is divided into overlapping regions of space.[96][97][98][99]
Near-Earth spaceis the region of space extending from low Earth orbits out togeostationary orbits.[96]This region includes the major orbits forartificial satellitesand is the site of most of humanity's space activity. The region has seen high levels of space debris, sometimes dubbedspace pollution,threatening any space activity in this region.[96]Some of this debris re-enters Earth's atmosphere periodically.[100]Although it meets the definition of outer space, the atmospheric density inside low-Earth orbital space, the first few hundred kilometers above the Kármán line, is still sufficient to produce significantdragon satellites.[95]
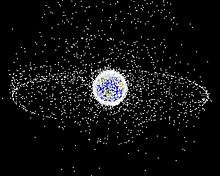
Geospaceis a region of space that includes Earth'supper atmosphereandmagnetosphere.[97]TheVan Allen radiation beltslie within the geospace. The outer boundary of geospace is themagnetopause,which forms an interface between the Earth's magnetosphere and the solar wind. The inner boundary is theionosphere.[102][103]
The variable space-weather conditions of geospace are affected by the behavior of the Sun and the solar wind; the subject of geospace is interlinked withheliophysics—the study of the Sun and its impact on the planets of the Solar System.[104]The day-side magnetopause is compressed by solar-wind pressure—the subsolar distance from the center of the Earth is typically 10 Earth radii. On the night side, the solar wind stretches the magnetosphere to form amagnetotailthat sometimes extends out to more than 100–200 Earth radii.[105][106]For roughly four days of each month, the lunar surface is shielded from the solar wind as the Moon passes through the magnetotail.[107]
Geospace is populated by electrically charged particles at very low densities, the motions of which are controlled by theEarth's magnetic field.These plasmas form a medium from which storm-like disturbances powered by the solar wind can drive electrical currents into the Earth's upper atmosphere.Geomagnetic stormscan disturb two regions of geospace, the radiation belts and the ionosphere. These storms increase fluxes of energetic electrons that can permanently damage satellite electronics, interfering with shortwave radio communication andGPSlocation and timing.[108]Magnetic storms can be a hazard to astronauts, even in low Earth orbit. They createauroraeseen at high latitudes in an oval surrounding thegeomagnetic poles.[109]

xGeo space is a concept used by the US to refer to space ofhigh Earth orbits,ranging from beyondgeosynchronous orbit(GEO) at approximately 35,786 km (22,236 mi),[98]out to theL2 Earth-Moon Lagrange pointat 448,900 km (278,934 mi). This is located beyond the orbit of the Moon and therefore includes cislunar space.[110]Translunar space is the region of lunartransfer orbits,between the Moon and Earth.[111]Cislunar spaceis a region outside of Earth that includeslunar orbits,theMoon's orbital space around Earthand theLagrange points.[99]
The region where a body'sgravitational potentialremains dominant against gravitational pontentials from other bodies, is the body'ssphere of influenceor gravity well, mostly described with theHill spheremodel.[112]In the case of Earth this includes all space from the Earth to a distance of roughly 1% of the mean distance from Earth to the Sun,[113]or 1.5 million km (0.93 million mi). Beyond Earth's Hill sphere extends alongEarth's orbitalpath its orbital andco-orbitalspace. This space is co-populated by groups of co-orbitalNear-Earth Objects(NEOs), such ashorseshoe libratorsandEarth trojans,with some NEOs at times becomingtemporary satellitesandquasi-moonsto Earth.[114]
Deep spaceis defined by the United States government as all of outer space which lies further from Earth than a typical low-Earth-orbit, thus assigning the moon to deep-space.[115]Other definitions vary the starting point of deep-space from, "That which lies beyond the orbit of the moon," to "That which lies beyond the farthest reaches of theSolar Systemitself. "[116][117][118]TheInternational Telecommunication Union responsible for radio communication,including with satellites, defines the beginning of deep-space as, "That which lies further than 2 million km (1.2 million mi) from Earth,[119]which is about five times theMoon's orbital distance,but which distance is also far less than the distance between Earth and any adjacent planet.[120]

Interplanetary space

Interplanetary space within theSolar Systemis the space between the eight planets, the space between the planets and the Sun, as well as that space beyond the orbit of the outermost planetNeptunewhere theSolar windremains active. The Solar wind is a continuous stream of charged particles emanating from the Sun which creates a very tenuous atmosphere (theheliosphere) for billions of kilometers into space. This wind has a particle density of 5–10protons/cm3and is moving at a velocity of 350–400 km/s (780,000–890,000 mph).[121]Interplanetary space extends out to theheliopausewhere the influence of the galactic environment starts to dominate over the magnetic field and particle flux from the Sun.[122]The distance and strength of the heliopause varies depending on the activity level of the solar wind.[123]The heliopause in turn deflects away low-energy galactic cosmic rays, with this modulation effect peaking during solar maximum.[124]
The volume of interplanetary space is a nearly total vacuum, with a mean free path of about oneastronomical unitat the orbital distance of the Earth. This space is not completely empty, and is sparsely filled with cosmic rays, which includeionizedatomic nucleiand various subatomic particles. There is gas, plasma and dust,[125]smallmeteors,and several dozen types oforganicmolecules discovered to date bymicrowave spectroscopy.[126]A cloud of interplanetary dust is visible at night as a faint band called thezodiacal light.[127]
Interplanetary space contains the magnetic field generated by the Sun.[121]There are magnetospheres generated by planets such as Jupiter, Saturn,Mercuryand the Earth that have their own magnetic fields. These are shaped by the influence of the solar wind into the approximation of a teardrop shape, with the long tail extending outward behind the planet. These magnetic fields can trap particles from the solar wind and other sources, creating belts of charged particles such as the Van Allen radiation belts. Planets without magnetic fields, such as Mars, have their atmospheres gradually eroded by the solar wind.[128]
Interstellar space

Interstellar space is the physical space outside of the bubbles of plasma known asastrospheres,formed bystellar windsoriginating from individual stars, or formed bySolar windemanating from the Sun.[129]It is the space between the stars orstellar systemswithin a nebula or galaxy.[130]Interstellar space contains aninterstellar mediumof sparse matter and radiation. The boundary between an astrosphere and interstellar space is known as anastropause.For the Sun, the astrosphere and astropause are called the heliosphere andheliopause.
Approximately 70% of the mass of the interstellar medium consists of lone hydrogen atoms; most of the remainder consists of helium atoms. This is enriched with trace amounts of heavier atoms formed throughstellar nucleosynthesis.These atoms are ejected into the interstellar medium by stellar winds or when evolved stars begin to shed their outer envelopes such as during the formation of aplanetary nebula.[131]The cataclysmic explosion of asupernovapropagatesshock wavesof stellar ejecta outward, distributing it throughout the interstellar medium, including the heavy elements previously formed within the star's core.[132]The density of matter in the interstellar medium can vary considerably: the average is around 106particles per m3,[133]but coldmolecular cloudscan hold 108–1012per m3.[39][131]
Anumber of moleculesexist in interstellar space, which can form dust particles as tiny as 0.1μm.[134]The tally of molecules discovered throughradio astronomyis steadily increasing at the rate of about four new species per year. Large regions of higher density matter known asmolecular cloudsallow chemical reactions to occur, including the formation of organic polyatomic species. Much of this chemistry is driven by collisions. Energetic cosmic rays penetrate the cold, dense clouds and ionize hydrogen and helium, resulting, for example, in thetrihydrogen cation.An ionized helium atom can then split relatively abundantcarbon monoxideto produce ionized carbon, which in turn can lead to organic chemical reactions.[135]
The local interstellar medium is a region of space within 100pcof the Sun, which is of interest both for its proximity and for its interaction with the Solar System. This volume nearly coincides with a region of space known as theLocal Bubble,which is characterized by a lack of dense, cold clouds. It forms a cavity in theOrion Armof the Milky Way galaxy, with dense molecular clouds lying along the borders, such as those in theconstellationsofOphiuchusandTaurus.(The actual distance to the border of this cavity varies from 60 to 250 pc or more.) This volume contains about 104–105stars and the local interstellar gas counterbalances theastrospheresthat surround these stars, with the volume of each sphere varying depending on the local density of the interstellar medium. The Local Bubble contains dozens of warm interstellar clouds with temperatures of up to 7,000 K and radii of 0.5–5 pc.[136]
When stars are moving at sufficiently highpeculiar velocities,their astrospheres can generatebow shocksas they collide with the interstellar medium. For decades it was assumed that the Sun had a bow shock. In 2012, data fromInterstellar Boundary Explorer (IBEX)and NASA'sVoyagerprobes showed that the Sun's bow shock does not exist. Instead, these authors argue that asubsonicbow wave defines the transition from the solar wind flow to the interstellar medium.[137][138]A bow shock is a third boundary characteristic of an astrosphere, laying outside thetermination shockand the astropause.[138]
Intergalactic space
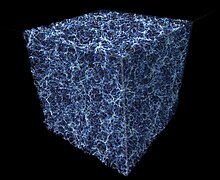
Intergalactic space is the physical space between galaxies. Studies of the large-scale distribution of galaxies show that the universe has a foam-like structure, withgroups and clusters of galaxieslying along filaments that occupy about a tenth of the total space. The remainder formscosmic voidsthat are mostly empty of galaxies. Typically, a void spans a distance of 7–30 megaparsecs.[139]
Surrounding and stretching between galaxies, there is ararefiedplasma[140]that is organized in agalactic filamentarystructure.[141]This material is called theintergalactic medium(IGM). The density of these filaments of intergalactic medium is about one atom per cubic meter,[142]which is 5–200 times the average density of the universe[143]after including the cosmic voids. The IGM is inferred to be mostly primordial in composition, with 76% hydrogen by mass, and enriched with higher mass elements from high-velocity galactic outflows.[144]
As gas falls into the intergalactic medium from the voids, it heats up to temperatures of 105K to 107K.[4]Hence, collisions between atoms have enough energy to cause the bound electron to escape from the hydrogen nuclei; this is why the IGM is ionized. At these temperatures, it is called thewarm–hot intergalactic medium(WHIM). (Although the plasma is very hot by terrestrial standards, 105K is often called "warm" in astrophysics.) Computer simulations and observations indicate that up to half of the atomic matter in the universe might exist in this warm–hot, rarefied state.[143][145][146]When gas falls from the filamentary structures of the WHIM into the galaxy clusters at the intersections of the cosmic filaments, it can heat up even more, reaching temperatures of 108K and above in the so-calledintracluster medium(ICM).[147]
History of discovery
In 350 BCE, Greek philosopherAristotlesuggested thatnature abhors a vacuum,a principle that became known as thehorror vacui.This concept built upon a 5th-century BCEontologicalargument by the Greek philosopherParmenides,who denied the possible existence of a void in space.[148]Based on this idea that a vacuum could not exist, in the West it was widely held for many centuries that space could not be empty.[149]As late as the 17th century, the French philosopherRené Descartesargued that the entirety of space must be filled.[150]
Inancient China,the 2nd-century astronomerZhang Hengbecame convinced that space must be infinite, extending well beyond the mechanism that supported the Sun and the stars. The surviving books of the Hsüan Yeh school said that the heavens were boundless, "empty and void of substance". Likewise, the "sun, moon, and the company of stars float in the empty space, moving or standing still".[151]
The Italian scientistGalileo Galileiknew that air had mass and so was subject to gravity. In 1640, he demonstrated that an established force resisted the formation of a vacuum. It would remain for his pupilEvangelista Torricellito create an apparatus that would produce a partial vacuum in 1643. This experiment resulted in the first mercurybarometerand created a scientific sensation in Europe. Torricelli suggested that since air has weight, thenair pressureshould decrease with altitude.[152]The French mathematicianBlaise Pascalproposed an experiment to test this hypothesis.[153]In 1648, his brother-in-law, Florin Périer, repeated the experiment on thePuy de Dômemountain in central France and found that the column was shorter by three inches. This decrease in pressure was further demonstrated by carrying a half-full balloon up a mountain and watching it gradually expand, then contract upon descent.[154]

In 1650, German scientistOtto von Guerickeconstructed the firstvacuum pump:a device that would further refute the principle ofhorror vacui.He correctly noted that the atmosphere of the Earth surrounds the planet like a shell, with the density gradually declining with altitude. He concluded that there must be a vacuum between the Earth and the Moon.[155]
In the 15th century, German theologianNicolaus Cusanusspeculated that the universe lacked a center and a circumference. He believed that the universe, while not infinite, could not be held as finite as it lacked any bounds within which it could be contained.[156]These ideas led to speculations as to the infinite dimension of space by the Italian philosopherGiordano Brunoin the 16th century. He extended the Copernicanheliocentriccosmology to the concept of an infinite universe filled with a substance he calledaether,which did not resist the motion of heavenly bodies.[157]English philosopherWilliam Gilbertarrived at a similar conclusion, arguing that the stars are visible to us only because they are surrounded by a thin aether or a void.[158]This concept of an aether originated with ancient Greek philosophers, including Aristotle, who conceived of it as the medium through which the heavenly bodies move.[159]
The concept of a universe filled with aluminiferous aetherretained support among some scientists until the early 20th century. This form of aether was viewed as the medium through which light could propagate.[160]In 1887, theMichelson–Morley experimenttried to detect the Earth's motion through this medium by looking for changes in thespeed of lightdepending on the direction of the planet's motion. Thenull resultindicated something was wrong with the concept. The idea of the luminiferous aether was then abandoned. It was replaced byAlbert Einstein's theory ofspecial relativity,which holds that the speed of light in a vacuum is a fixed constant, independent of the observer's motion orframe of reference.[161][162]
The first professional astronomer to support the concept of an infinite universe was the EnglishmanThomas Diggesin 1576.[163]But the scale of the universe remained unknown until the first successful measurement of the distance to a nearby star in 1838 by the German astronomerFriedrich Bessel.He showed that the star system61 Cygnihad aparallaxof just 0.31arcseconds(compared to the modern value of 0.287″). This corresponds to a distance of over 10light years.[164]In 1917,Heber Curtisnoted thatnovaein spiral nebulae were, on average, 10 magnitudes fainter than galactic novae, suggesting that the former are 100 times further away.[165]The distance to theAndromeda Galaxywas determined in 1923 by American astronomerEdwin Hubbleby measuring the brightness ofcepheid variablesin that galaxy, a new technique discovered byHenrietta Leavitt.[166]This established that the Andromeda galaxy, and by extension all galaxies, lay well outside the Milky Way.[167]
The modern concept of outer space is based on the"Big Bang" cosmology,first proposed in 1931 by the Belgian physicistGeorges Lemaître.[168]This theory holds that the universe originated from a state of extremeenergy densitythat has since undergonecontinuous expansion.[169]
The earliest known estimate of the temperature of outer space was by the Swiss physicistCharles É. Guillaumein 1896. Using the estimated radiation of the background stars, he concluded that space must be heated to a temperature of 5–6 K. British physicistArthur Eddingtonmade a similar calculation to derive a temperature of 3.18 K in 1926. German physicistErich Regenerused the total measured energy ofcosmic raysto estimate an intergalactic temperature of 2.8 K in 1933.[170]American physicistsRalph AlpherandRobert Hermanpredicted 5 K for the temperature of space in 1948, based on the gradual decrease in background energy following the then-newBig Bangtheory.[170]
Exploration

For most of human history, space was explored by observations made from the Earth's surface—initially with the unaided eye and then with the telescope. Before reliable rocket technology, the closest that humans had come to reaching outer space was through balloon flights. In 1935, the AmericanExplorer IIcrewed balloon flight reached an altitude of 22 km (14 mi).[172]This was greatly exceeded in 1942 when the third launch of the GermanA-4 rocketclimbed to an altitude of about 80 km (50 mi). In 1957, the uncrewed satelliteSputnik 1was launched by a RussianR-7 rocket,achieving Earth orbit at an altitude of 215–939 kilometres (134–583 mi).[173]This was followed by the first human spaceflight in 1961, whenYuri Gagarinwas sent into orbit onVostok 1.The first humans to escape low Earth orbit wereFrank Borman,Jim LovellandWilliam Andersin 1968 on board the AmericanApollo 8,which achieved lunar orbit[174]and reached a maximum distance of 377,349 km (234,474 mi) from the Earth.[175]
The first spacecraft to reach escape velocity was the SovietLuna 1,which performed a fly-by of the Moon in 1959.[176]In 1961,Venera 1became the first planetary probe. It revealed the presence of the solar wind and performed the first fly-by ofVenus,although contact was lost before reaching Venus. The first successful planetary mission was the 1962 fly-by of Venus byMariner 2.[177]The first fly-by of Mars was byMariner 4in 1964. Since that time, uncrewed spacecraft have successfully examined each of the Solar System's planets, as well their moons and manyminor planetsand comets. They remain a fundamental tool for the exploration of outer space, as well as for observation of the Earth.[178]In August 2012,Voyager 1became the first man-made object to leave the Solar System and enterinterstellar space.[179]
Application

Outer space has become an important element of global society. It provides multiple applications that are beneficial to the economy and scientific research.
The placing of artificial satellites in Earth orbit has produced numerous benefits and has become the dominating sector of thespace economy.They allow relay oflong-range communicationslike television, provide a means ofprecise navigation,and permit direct monitoring ofweather conditionsandremote sensingof the Earth. The latter role serves a variety of purposes, including tracking soil moisture for agriculture, prediction of water outflow from seasonal snow packs, detection of diseases in plants and trees, andsurveillanceof military activities.[181]They facilitate the discovery and monitoring ofclimate changeinfluences.[182]Satellites make use of the significantly reduced drag in space to stay in stable orbits, allowing them to efficiently span the whole globe, compared to for examplestratospheric balloonsorhigh-altitude platform stations,which have other benefits.[183]
The absence of air makes outer space an ideal location for astronomy at all wavelengths of theelectromagnetic spectrum.This is evidenced by the spectacular pictures sent back by theHubble Space Telescope,allowing light from more than 13 billion years ago—almost to the time of the Big Bang—to be observed.[184]Not every location in space is ideal for a telescope. Theinterplanetary zodiacal dustemits a diffuse near-infrared radiation that can mask the emission of faint sources such as extrasolar planets. Moving aninfrared telescopeout past the dust increases its effectiveness.[185]Likewise, a site like theDaedalus crateron thefar side of the Mooncould shield aradio telescopefrom theradio frequency interferencethat hampers Earth-based observations.[186]

The deep vacuum of space could make it an attractive environment for certain industrial processes, such as those requiring ultraclean surfaces.[187]Likeasteroid mining,space manufacturingwould require a large financial investment with little prospect of immediate return.[188]An important factor in the total expense is the high cost of placing mass into Earth orbit: $9,000–$30,000 per kg, according to a 2006 estimate (allowing for inflation since then).[189]The cost of access to space has declined since 2013. Partially reusable rockets such as theFalcon 9have lowered access to space below 3500 dollars per kilogram. With these new rockets the cost to send materials into space remains prohibitively high for many industries. Proposed concepts for addressing this issue include, fullyreusable launch systems,non-rocket spacelaunch,momentum exchange tethers,andspace elevators.[190]
Interstellar travelfor a human crew remains at present only a theoretical possibility. The distances to the nearest stars mean it would require new technological developments and the ability to safely sustain crews for journeys lasting several decades. For example, theDaedalus Projectstudy, which proposed a spacecraft powered by thefusionofdeuteriumandhelium-3,would require 36 years to reach the "nearby"Alpha Centaurisystem. Other proposed interstellar propulsion systems includelight sails,ramjets,andbeam-powered propulsion.More advanced propulsion systems could useantimatteras a fuel, potentially reachingrelativistic velocities.[191]
From the Earth's surface, the ultracold temperature of outer space can be used as arenewablecooling technology for various applications on Earth throughpassive daytime radiative cooling.[192][193]This enhanceslongwave infrared(LWIR)thermal radiationheat transfer through the amosphere'sinfrared windowinto outer space, lowering ambient temperatures.[194][195]Photonic metamaterialscan be used to suppress solar heating.[196]
See also
- Absolute space and time
- List of government space agencies
- List of topics in space
- Olbers' paradox
- Outline of space science
- Panspermia
- Space art
- Space and survival
- Space race
- Space station
- Space technology
- Timeline of knowledge about the interstellar and intergalactic medium
- Timeline of Solar System exploration
- Timeline of spaceflight
References
Citations
- ^"Applicable definitions of outer space, space, and expanse",Merriam-Webster dictionary,retrieved2024-06-17,
Outer space(n.) space immediately outside the earth's atmosphere.
Space(n.) physical space independent of what occupies it. The region beyond the earth's atmosphere or beyond the solar system.
Expanse(n.) great extent of something spread out. - ^Roth, A. (2012),Vacuum Technology,Elsevier, p. 6,ISBN978-0-444-59874-5.
- ^Chuss, David T. (June 26, 2008),Cosmic Background Explorer,NASA Goddard Space Flight Center,archivedfrom the original on May 9, 2013,retrieved2013-04-27.
- ^abGupta, Anjali; et al. (May 2010), "Detection and Characterization of the Warm-Hot Intergalactic Medium",Bulletin of the American Astronomical Society,41:908,Bibcode:2010AAS...21631808G.
- ^Freedman & Kaufmann 2005,pp. 573, 599–601.
- ^Trimble, V. (1987),"Existence and nature of dark matter in the universe",Annual Review of Astronomy and Astrophysics,25:425–472,Bibcode:1987ARA&A..25..425T,doi:10.1146/annurev.aa.25.090187.002233,S2CID123199266.
- ^"Dark Energy, Dark Matter",NASA Science,archivedfrom the original on June 2, 2013,retrievedMay 31,2013,
It turns out that roughly 68% of the Universe is dark energy. Dark matter makes up about 27%.
- ^Freedman & Kaufmann 2005,pp. 650–653.
- ^abO'Leary 2009,p. 84.
- ^ab"Where does space begin?",Aerospace Engineering,archivedfrom the original on 2015-11-17,retrieved2015-11-10.
- ^Harper, Douglas (November 2001),Space,The Online Etymology Dictionary,archivedfrom the original on 2009-02-24,retrieved2009-06-19.
- ^Brady, Maura (October 2007), "Space and the Persistence of Place in" Paradise Lost "",Milton Quarterly,41(3): 167–182,doi:10.1111/j.1094-348X.2007.00164.x,JSTOR24461820.
- ^Stuart Wortley 1841,p. 410.
- ^Von Humboldt 1845,p. 39.
- ^Harper, Douglas,"Outer",Online Etymology Dictionary,archivedfrom the original on 2010-03-12,retrieved2008-03-24.
- ^abcdBetz, Eric (2023-11-27)."The Kármán Line: Where space begins".Astronomy Magazine.Retrieved2024-04-30.
- ^"Definition of SPACEBORNE",Merriam-Webster,2022-05-17,retrieved2022-05-18.
- ^"Spaceborne definition and meaning",Collins English Dictionary,2022-05-17,retrieved2022-05-18.
- ^"-based",Cambridge Dictionary,2024,retrieved2024-04-28.
- ^Liddle 2015,pp. 33.
- ^Planck Collaboration (2014), "Planck 2013 results. I. Overview of products and scientific results",Astronomy & Astrophysics,571:1,arXiv:1303.5062,Bibcode:2014A&A...571A...1P,doi:10.1051/0004-6361/201321529,S2CID218716838.
- ^abTurner, Michael S. (September 2009), "Origin of the Universe",Scientific American,301(3): 36–43,Bibcode:2009SciAm.301c..36T,doi:10.1038/scientificamerican0909-36,PMID19708526.
- ^Silk 2000,pp. 105–308.
- ^WMAP – Shape of the universe,NASA, December 21, 2012,archivedfrom the original on June 1, 2012,retrievedJune 4,2013.
- ^Sparke & Gallagher 2007,pp. 329–330.
- ^Wollack, Edward J. (June 24, 2011),What is the Universe Made Of?,NASA,archivedfrom the original on July 26, 2016,retrieved2011-10-14.
- ^Krumm, N.; Brosch, N. (October 1984), "Neutral hydrogen in cosmic voids",Astronomical Journal,89:1461–1463,Bibcode:1984AJ.....89.1461K,doi:10.1086/113647.
- ^Peebles, P.; Ratra, B. (2003), "The cosmological constant and dark energy",Reviews of Modern Physics,75(2): 559–606,arXiv:astro-ph/0207347,Bibcode:2003RvMP...75..559P,doi:10.1103/RevModPhys.75.559,S2CID118961123
- ^"False Dawn",www.eso.org,retrieved14 February2017.
- ^Tadokoro, M. (1968), "A Study of the Local Group by Use of the Virial Theorem",Publications of the Astronomical Society of Japan,20:230,Bibcode:1968PASJ...20..230T.This source estimates a density of7 × 10−29g/cm3for theLocal Group.Anatomic mass unitis1.66 × 10−24g,for roughly 40 atoms per cubic meter.
- ^Borowitz & Beiser 1971.
- ^Tyson, Patrick (January 2012),The Kinetic Atmosphere: Molecular Numbers(PDF),archived fromthe original(PDF)on 7 December 2013,retrieved13 September2013.
- ^Davies 1977,p. 93.
- ^Fitzpatrick, E. L. (May 2004), "Interstellar Extinction in the Milky Way Galaxy", in Witt, Adolf N.; Clayton, Geoffrey C.; Draine, Bruce T. (eds.),Astrophysics of Dust,ASP Conference Series, vol. 309, p. 33,arXiv:astro-ph/0401344,Bibcode:2004ASPC..309...33F.
- ^Chamberlain 1978,p. 2.
- ^Squire, Tom (September 27, 2000),"U.S. Standard Atmosphere, 1976",Thermal Protection Systems Expert and Material Properties Database,NASA, archived fromthe originalon October 15, 2011,retrieved2011-10-23.
- ^Forbes, Jeffrey M. (2007), "Dynamics of the thermosphere",Journal of the Meteorological Society of Japan,Series II,85B:193–213,Bibcode:2007JMeSJ..85B.193F,doi:10.2151/jmsj.85b.193.
- ^Spitzer, Lyman Jr.(January 1948), "The Temperature of Interstellar Matter. I",Astrophysical Journal,107:6,Bibcode:1948ApJ...107....6S,doi:10.1086/144984.
- ^abPrialnik 2000,pp. 195–196.
- ^Spitzer 1978,p. 28–30.
- ^Chiaki, Yanagisawa (June 2014), "Looking for Cosmic Neutrino Background",Frontiers in Physics,2:30,Bibcode:2014FrP.....2...30Y,doi:10.3389/fphy.2014.00030.
- ^Fixsen, D. J. (December 2009), "The Temperature of the Cosmic Microwave Background",The Astrophysical Journal,707(2): 916–920,arXiv:0911.1955,Bibcode:2009ApJ...707..916F,doi:10.1088/0004-637X/707/2/916,S2CID119217397.
- ^ALMA reveals ghostly shape of 'coldest place in the universe',National Radio Astronomy Observatory, October 24, 2013,retrieved2020-10-07.
- ^Withbroe, George L. (February 1988), "The temperature structure, mass, and energy flow in the corona and inner solar wind",Astrophysical Journal, Part 1,325:442–467,Bibcode:1988ApJ...325..442W,doi:10.1086/166015.
- ^Wielebinski, Richard; Beck, Rainer (2010), "Cosmic Magnetic Fields − An Overview", in Block, David L.; Freeman, Kenneth C.; Puerari, Ivânio (eds.),Galaxies and their Masks: A Conference in Honour of K.C. Freeman, FRS,Springer Science & Business Media, pp. 67–82,Bibcode:2010gama.conf...67W,doi:10.1007/978-1-4419-7317-7_5,ISBN978-1-4419-7317-7,archivedfrom the original on 2017-09-20.
- ^Letessier-Selvon, Antoine; Stanev, Todor (July 2011), "Ultrahigh energy cosmic rays",Reviews of Modern Physics,83(3): 907–942,arXiv:1103.0031,Bibcode:2011RvMP...83..907L,doi:10.1103/RevModPhys.83.907,S2CID119237295.
- ^Lang 1999,p. 462.
- ^Lide 1993,p. 11-217.
- ^What Does Space Smell Like?,Live Science, July 20, 2012,archivedfrom the original on February 28, 2014,retrievedFebruary 19,2014.
- ^Lizzie Schiffman (July 17, 2013),What Does Space Smell Like,Popular Science,archivedfrom the original on February 24, 2014,retrievedFebruary 19,2014.
- ^Raggio, J.; et al. (May 2011), "Whole Lichen Thalli Survive Exposure to Space Conditions: Results of Lithopanspermia Experiment with Aspicilia fruticulosa",Astrobiology,11(4): 281–292,Bibcode:2011AsBio..11..281R,doi:10.1089/ast.2010.0588,PMID21545267.
- ^Tepfer, David; et al. (May 2012),"Survival of Plant Seeds, Their UV Screens, and nptII DNA for 18 Months Outside the International Space Station"(PDF),Astrobiology,12(5): 517–528,Bibcode:2012AsBio..12..517T,doi:10.1089/ast.2011.0744,PMID22680697,archived(PDF)from the original on 2014-12-13,retrieved2013-05-19.
- ^Wassmann, Marko; et al. (May 2012), "Survival of Spores of the UV-ResistantBacillus subtilis Strain MW01 After Exposure to Low-Earth Orbit and Simulated Martian Conditions: Data from the Space Experiment ADAPT on EXPOSE-E",Astrobiology,12(5): 498–507,Bibcode:2012AsBio..12..498W,doi:10.1089/ast.2011.0772,PMID22680695.
- ^Nicholson, W. L. (April 2010), "Towards a General Theory of Lithopanspermia",Astrobiology Science Conference 2010,vol. 1538, pp. 5272–528,Bibcode:2010LPICo1538.5272N.
- ^Tarver, William J.; et al. (October 24, 2022),Aerospace Pressure Effects,Treasure Island, FL: StatPearls Publishing,PMID29262037,retrieved2024-04-25.
- ^Piantadosi 2003,pp. 188–189.
- ^Battisti, Amanda S.; et al. (June 27, 2022),Barotrauma,StatPearls Publishing LLC,PMID29493973,retrieved2022-12-18.
- ^Krebs, Matthew B.; Pilmanis, Andrew A. (November 1996),Human pulmonary tolerance to dynamic over-pressure(PDF),United States Air Force Armstrong Laboratory,archivedfrom the original on 2012-11-30,retrieved2011-12-23.
- ^Busby, D. E. (July 1967),A prospective look at medical problems from hazards of space operations(PDF),Clinical Space Medicine, NASA, NASA-CR-856,retrieved2022-12-20.
- ^Harding, R. M.; Mills, F. J. (April 30, 1983), "Aviation medicine. Problems of altitude I: hypoxia and hyperventilation",British Medical Journal,286(6375): 1408–1410,doi:10.1136/bmj.286.6375.1408,PMC1547870,PMID6404482.
- ^Hodkinson, P. D. (March 2011),"Acute exposure to altitude"(PDF),Journal of the Royal Army Medical Corps,157(1): 85–91,doi:10.1136/jramc-157-01-15,PMID21465917,S2CID43248662,archived fromthe original(PDF)on 2012-04-26,retrieved2011-12-16.
- ^Billings 1973,pp. 1–34.
- ^Landis, Geoffrey A. (August 7, 2007),Human Exposure to Vacuum,www.geoffreylandis.com, archived fromthe originalon July 21, 2009,retrieved2009-06-19.
- ^Webb, P. (1968), "The Space Activity Suit: An Elastic Leotard for Extravehicular Activity",Aerospace Medicine,39(4): 376–383,PMID4872696.
- ^Ellery 2000,p. 68.
- ^Davis, Johnson & Stepanek 2008,pp. 270–271.
- ^Kanas & Manzey 2008,pp. 15–48.
- ^Williams, David; et al. (June 23, 2009), "Acclimation during space flight: effects on human physiology",Canadian Medical Association Journal,180(13): 1317–1323,doi:10.1503/cmaj.090628,PMC2696527,PMID19509005.
- ^Kennedy, Ann R.,Radiation Effects,National Space Biological Research Institute,archivedfrom the original on 2012-01-03,retrieved2011-12-16.
- ^Curtis, S. B.; Letaw, J. W. (1989), "Galactic cosmic rays and cell-hit frequencies outside the magnetosphere",Advances in Space Research,9(10): 293–298,Bibcode:1989AdSpR...9c.293C,doi:10.1016/0273-1177(89)90452-3,PMID11537306
- ^Setlow, Richard B. (November 2003), "The hazards of space travel",Science and Society,4(11): 1013–1016,doi:10.1038/sj.embor.7400016,PMC1326386,PMID14593437.
- ^abcGrush, Loren (2018-12-13)."Why defining the boundary of space may be crucial for the future of spaceflight".The Verge.Retrieved2024-04-30.
- ^Wong & Fergusson 2010,p. 16.
- ^FAA Commercial Space Astronaut Wings Program(PDF),Federal Aviation Administration, July 20, 2021,retrieved2022-12-18.
- ^Thompson, Andrea (April 9, 2009),Edge of Space Found,space.com,archivedfrom the original on July 14, 2009,retrieved2009-06-19.
- ^Sangalli, L.; et al. (2009), "Rocket-based measurements of ion velocity, neutral wind, and electric field in the collisional transition region of the auroral ionosphere",Journal of Geophysical Research,114(A4): A04306,Bibcode:2009JGRA..114.4306S,doi:10.1029/2008JA013757.
- ^abcMcDowell, Jonathan C. (October 2018), "The edge of space: Revisiting the Karman Line",Acta Astronautica,151:668–677,arXiv:1807.07894,Bibcode:2018AcAau.151..668M,doi:10.1016/j.actaastro.2018.07.003.
- ^Petty, John Ira (February 13, 2003),"Entry",Human Spaceflight,NASA, archived fromthe originalon October 27, 2011,retrieved2011-12-16.
- ^Durrani, Haris (19 July 2019),"Is Spaceflight Colonialism?",The Nation,retrieved6 October2020.
- ^Status of International Agreements relating to activities in outer space as of 1 January 2017(PDF),United Nations Office for Outer Space Affairs/ Committee on the Peaceful Uses of Outer Space, March 23, 2017, archived fromthe original(PDF)on March 22, 2018,retrieved2018-03-22.
- ^Treaty on Principles Governing the Activities of States in the Exploration and Use of Outer Space, including the Moon and Other Celestial Bodies,United Nations Office for Outer Space Affairs, January 1, 2008, archived fromthe originalon April 27, 2011,retrieved2009-12-30.
- ^Index of Online General Assembly Resolutions Relating to Outer Space,United Nations Office for Outer Space Affairs, 2011,archivedfrom the original on 2010-01-15,retrieved2009-12-30.
- ^Wong & Fergusson 2010,p. 4.
- ^Solanki, Lalit (2019-03-27),"India Enters the Elite Club: Successfully Shot Down Low Orbit Satellite",The Mirk,retrieved2019-03-28.
- ^Columbus launch puts space law to the test,European Science Foundation, November 5, 2007, archived fromthe originalon December 15, 2008,retrieved2009-12-30.
- ^Representatives of the States traversed by the Equator (December 3, 1976),"Declaration of the first meeting of equatorial countries",Space Law,Bogota, Republic of Colombia: JAXA,archivedfrom the original on November 24, 2011,retrieved2011-10-14.
- ^Gangale, Thomas (2006),"Who Owns the Geostationary Orbit?",Annals of Air and Space Law,31,archived fromthe originalon 2011-09-27,retrieved2011-10-14.
- ^"ESIL Reflection – Clearing up the Space Junk – On the Flaws and Potential of International Space Law to Tackle the Space Debris Problem – European Society of International Law",European Society of International Law,2023-03-09,retrieved2024-04-24.
- ^Hill, James V. H. (April 1999),"Getting to Low Earth Orbit",Space Future,archivedfrom the original on 2012-03-19,retrieved2012-03-18.
- ^Shiner, Linda (November 1, 2007),X-15 Walkaround,Air & Space Magazine,retrieved2009-06-19.
- ^Dimotakis, P.; et al. (October 1999),100 lbs to Low Earth Orbit (LEO): Small-Payload Launch Options,The Mitre Corporation, pp. 1–39, archived fromthe originalon 2017-08-29,retrieved2012-01-21.
- ^Williams, David R. (November 17, 2010),"Earth Fact Sheet",Lunar & Planetary Science,NASA,archivedfrom the original on October 30, 2010,retrieved2012-05-10.
- ^Ghosh 2000,pp. 47–48.
- ^Frequently Asked Questions,Astromaterials Research & Exploration Science: NASA Orbital Debris Program Office,retrieved2024-04-29.
- ^abKennewell, John; McDonald, Andrew (2011),Satellite Lifetimes and Solar Activity,Commonwealth of Australia Bureau of Weather, Space Weather Branch,archivedfrom the original on 2011-12-28,retrieved2011-12-31.
- ^abc"42 USC 18302: Definitions",uscode.house.gov(in Kinyarwanda), December 15, 2022,retrievedDecember 17,2022.
- ^abSchrijver & Siscoe 2010,p. 363, 379.
- ^abHowell, Elizabeth (April 24, 2015),"What Is a Geosynchronous Orbit?",Space.com,retrieved8 December2022.
- ^abStrickland, John K. (October 1, 2012),The cislunar gateway with no gate,The Space Review,archivedfrom the original on February 7, 2016,retrieved2016-02-10.
- ^Portree, David; Loftus, Joseph (1999),"Orbital Debris: A Chronology"(PDF),NASA Sti/Recon Technical Report N,99,NASA: 13,Bibcode:1999STIN...9941786P,archived fromthe original(PDF)on 2000-09-01,retrieved2012-05-05.
- ^Photo Gallery,ARES | NASA Orbital Debris Program Office,retrieved2024-04-27.
- ^Kintner, Paul; GMDT Committee and Staff (September 2002),Report of the Living With a Star Geospace Mission Definition Team(PDF),NASA,archived(PDF)from the original on 2012-11-02,retrieved2012-04-15.
- ^Schrijver & Siscoe 2010,p. 379.
- ^Fichtner & Liu 2011,pp. 341–345.
- ^Koskinen 2010,pp. 32, 42.
- ^Hones, Edward W. Jr. (March 1986), "The Earth's Magnetotail",Scientific American,254(3): 40–47,Bibcode:1986SciAm.254c..40H,doi:10.1038/scientificamerican0386-40,JSTOR24975910
- ^Mendillo 2000,p. 275.
- ^Goodman 2006,p. 244.
- ^"Geomagnetic Storms"(PDF),OECD/IFP Futures Project on "Future Global Shocks",CENTRA Technology, Inc., pp. 1–69, January 14, 2011,archived(PDF)from the original on March 14, 2012,retrieved2012-04-07.
- ^Hitchens, Theresa (2022-04-21),"To infinity and beyond: New Space Force unit to monitor 'xGEO' beyond Earth's orbit",Breaking Defense,retrieved2022-12-17.
- ^"Why We Explore",NASA,June 13, 2013,retrievedDecember 17,2022.
- ^Yoder, Charles F. (1995), "Astrometric and Geodetic Properties of Earth and the Solar System", in Ahrens, Thomas J. (ed.),Global earth physics a handbook of physical constants(PDF),AGU reference shelf Series, vol. 1, Washington, DC: American Geophysical Union, p. 1,Bibcode:1995geph.conf....1Y,ISBN978-0-87590-851-9,archived fromthe original(PDF)on April 26, 2012,retrieved2011-12-31..This work lists a Hill sphere radius of 234.9 times themean radiusof Earth, or 234.9 × 6,371 km = 1.5 million km.
- ^Barbieri 2006,p. 253.
- ^Granvik, Mikael; et al. (March 2012), "The population of natural Earth satellites",Icarus,218(1): 262–277,arXiv:1112.3781,Bibcode:2012Icar..218..262G,doi:10.1016/j.icarus.2011.12.003.
- ^"51 U.S.C 10101 -National and Commercial Space Programs, Subtitle I-General, Chapter 101-Definitions",United States Code,Office of Law Revision Council, U. S. House of Representatives,retrievedJanuary 5,2023.
- ^Dickson 2010,p. 57.
- ^Williamson 2006,p. 97.
- ^"Definition of 'deep space'",Collins English Dictionary,retrieved2018-01-15.
- ^ITU-R Radio Regulations, Article 1, Terms and definitions, Section VIII, Technical terms relating to space, paragraph 1.177.(PDF),International Telecommunication Union,retrieved2018-02-05.
- ^The semi-major axis of the Moon's orbit is384,400 km,which is 19.2% of two million km, or about one-fifth.
Williams, David R. (December 20, 2021),Moon Fact Sheet,NASA,retrieved2023-09-23. - ^abPapagiannis 1972,pp. 12–149.
- ^Abby Cessna (July 5, 2009),"Interplanetary space",Universe Today,archivedfrom the original on March 19, 2015.
- ^Phillips, Tony (2009-09-29),Cosmic Rays Hit Space Age High,NASA, archived fromthe originalon 2009-10-14,retrieved2009-10-20.
- ^Kohler, Susanna (December 1, 2017),"A Shifting Shield Provides Protection Against Cosmic Rays",Nova,American Astronomical Society, p. 2992,Bibcode:2017nova.pres.2992K,retrieved2019-01-31.
- ^NASA (March 12, 2019),"What scientists found after sifting through dust in the solar system",EurekAlert!,retrieved12 March2019.
- ^Flynn, G. J.; et al. (2003), "The Origin of Organic Matter in the Solar System: Evidence from the Interplanetary Dust Particles", in Norris, R.; Stootman, F. (eds.),Bioastronomy 2002: Life Among the Stars, Proceedings of IAU Symposium No. 213,vol. 213, p. 275,Bibcode:2004IAUS..213..275F.
- ^Leinert, C.; Grun, E. (1990), "Interplanetary Dust",Physics of the Inner Heliosphere I,p. 207,Bibcode:1990pihl.book..207L,doi:10.1007/978-3-642-75361-9_5,ISBN978-3-642-75363-3.
- ^Johnson, R. E. (August 1994), "Plasma-Induced Sputtering of an Atmosphere",Space Science Reviews,69(3–4): 215–253,Bibcode:1994SSRv...69..215J,doi:10.1007/BF02101697,S2CID121800711.
- ^Jia-Rui Cook (September 12, 2013),"How do we know when Voyager reaches interstellar space?",JPL News,2013-278,archivedfrom the original on September 15, 2013.
- ^Cooper, Keith (2023-01-17)."Interstellar space: What is it and where does it begin?".Space.com.Retrieved2024-01-30.
- ^abFerrière, Katia M. (2001), "The interstellar environment of our galaxy",Reviews of Modern Physics,73(4): 1031–1066,arXiv:astro-ph/0106359,Bibcode:2001RvMP...73.1031F,doi:10.1103/RevModPhys.73.1031,S2CID16232084.
- ^Witt, Adolf N. (October 2001), "The Chemical Composition of the Interstellar Medium",Philosophical Transactions of the Royal Society A: Mathematical, Physical and Engineering Sciences – Origin and early evolution of solid matter in the Solar System,vol. 359, p. 1949,Bibcode:2001RSPTA.359.1949W,doi:10.1098/rsta.2001.0889,S2CID91378510.
- ^Boulares, Ahmed; Cox, Donald P. (December 1990), "Galactic hydrostatic equilibrium with magnetic tension and cosmic-ray diffusion",Astrophysical Journal, Part 1,365:544–558,Bibcode:1990ApJ...365..544B,doi:10.1086/169509.
- ^Rauchfuss 2008,pp. 72–81.
- ^Klemperer, William (August 15, 2006), "Interstellar chemistry",Proceedings of the National Academy of Sciences of the United States of America,103(33): 12232–12234,Bibcode:2006PNAS..10312232K,doi:10.1073/pnas.0605352103,PMC1567863,PMID16894148.
- ^Redfield, S. (September 2006), "The Local Interstellar Medium",New Horizons in Astronomy; Proceedings of the Conference Held 16–18 October 2005 at The University of Texas, Austin, Texas, USA,Frank N. Bash Symposium ASP Conference Series, vol. 352, p. 79,arXiv:astro-ph/0601117,Bibcode:2006ASPC..352...79R.
- ^McComas, D. J.; et al. (2012), "The Heliosphere's Interstellar Interaction: No Bow Shock",Science,336(6086): 1291–3,Bibcode:2012Sci...336.1291M,doi:10.1126/science.1221054,PMID22582011,S2CID206540880.
- ^abFox, Karen C. (May 10, 2012),NASA – IBEX Reveals a Missing Boundary at the Edge of the Solar System,NASA,archivedfrom the original on May 12, 2012,retrieved2012-05-14.
- ^Wszolek 2013,p. 67.
- ^Jafelice, Luiz C.; Opher, Reuven (July 1992), "The origin of intergalactic magnetic fields due to extragalactic jets",Monthly Notices of the Royal Astronomical Society,257(1): 135–151,Bibcode:1992MNRAS.257..135J,doi:10.1093/mnras/257.1.135.
- ^Wadsley, James W.; et al. (August 20, 2002),"The Universe in Hot Gas",Astronomy Picture of the Day,NASA,archivedfrom the original on June 9, 2009,retrieved2009-06-19.
- ^"Intergalactic medium",Harvard & Smithsonian,2022-06-16,retrieved2024-04-16.
- ^abFang, T.; et al. (2010), "Confirmation of X-Ray Absorption by Warm-Hot Intergalactic Medium in the Sculptor Wall",The Astrophysical Journal,714(2): 1715,arXiv:1001.3692,Bibcode:2010ApJ...714.1715F,doi:10.1088/0004-637X/714/2/1715,S2CID17524108.
- ^Oppenheimer, Benjamin D.; Davé, Romeel (December 2006), "Cosmological simulations of intergalactic medium enrichment from galactic outflows",Monthly Notices of the Royal Astronomical Society,373(4): 1265–1292,arXiv:astro-ph/0605651,Bibcode:2006MNRAS.373.1265O,doi:10.1111/j.1365-2966.2006.10989.x.
- ^Bykov, A. M.; et al. (February 2008), "Equilibration Processes in the Warm-Hot Intergalactic Medium",Space Science Reviews,134(1–4): 141–153,arXiv:0801.1008,Bibcode:2008SSRv..134..141B,doi:10.1007/s11214-008-9309-4,S2CID17801881.
- ^Wakker, B. P.; Savage, B. D. (2009), "The Relationship Between Intergalactic H I/O VI and Nearby (z<0.017) Galaxies",The Astrophysical Journal Supplement Series,182(1): 378,arXiv:0903.2259,Bibcode:2009ApJS..182..378W,doi:10.1088/0067-0049/182/1/378,S2CID119247429.
- ^Mathiesen, B. F.; Evrard, A. E. (2001), "Four Measures of the Intracluster Medium Temperature and Their Relation to a Cluster's Dynamical State",The Astrophysical Journal,546(1): 100,arXiv:astro-ph/0004309,Bibcode:2001ApJ...546..100M,doi:10.1086/318249,S2CID17196808.
- ^Grant 1981,p. 10.
- ^Porter, Park & Daston 2006,p. 27.
- ^Eckert 2006,p. 5.
- ^Needham & Ronan 1985,pp. 82–87.
- ^West, John B. (March 2013), "Torricelli and the Ocean of Air: The First Measurement of Barometric Pressure",Physiology (Bethesda),28(2): 66–73,doi:10.1152/physiol.00053.2012,PMC3768090,PMID23455767.
- ^Holton & Brush 2001,pp. 267–268.
- ^Cajori 1917,pp. 64–66.
- ^Genz 2001,pp. 127–128.
- ^Tassoul & Tassoul 2004,p. 22.
- ^Gatti 2002,pp. 99–104.
- ^Kelly 1965,pp. 97–107.
- ^Olenick, Apostol & Goodstein 1986,p. 356.
- ^Hariharan 2003,p. 2.
- ^Olenick, Apostol & Goodstein 1986,pp. 357–365.
- ^Thagard 1992,pp. 206–209.
- ^Maor 1991,p. 195.
- ^Webb 1999,pp. 71–73.
- ^Curtis, Heber D. (January 1988), "Novae in Spiral Nebulae and the Island Universe Theory",Publications of the Astronomical Society of the Pacific,100:6–7,Bibcode:1988PASP..100....6C,doi:10.1086/132128.
- ^Cepheid Variable Stars & Distance Determination,CSIRO Australia, October 25, 2004,archivedfrom the original on August 30, 2011,retrieved2011-09-12.
- ^Tyson & Goldsmith 2004,pp. 114–115.
- ^Lemaître, G.(May 1931), "The Beginning of the World from the Point of View of Quantum Theory",Nature,127(3210): 706,Bibcode:1931Natur.127..706L,doi:10.1038/127706b0,S2CID4089233.
- ^Big Bang Cosmology,NASA,retrieved2024-04-24.
- ^abAssis, A. K. T.; et al. (July 1995), "History of the 2.7 K Temperature Prior to Penzias and Wilson",Apeiron,2(3): 79–87.
- ^Woods, W. David; O'Brien, Frank (2006),"Day 1: The Green Team and Separation",Apollo 8 Flight Journal,NASA, archived fromthe originalon September 23, 2008,retrievedOctober 29,2008.TIMETAG 003:42:55.
- ^Pfotzer, G. (June 1972), "History of the Use of Balloons in Scientific Experiments",Space Science Reviews,13(2): 199–242,Bibcode:1972SSRv...13..199P,doi:10.1007/BF00175313,S2CID120710485.
- ^O'Leary 2009,pp. 209–224.
- ^Harrison 2002,pp. 60–63.
- ^Orloff 2001.
- ^Hardesty, Eisman & Krushchev 2008,pp. 89–90.
- ^Collins 2007,p. 86.
- ^Harris 2008,pp. 7, 68–69.
- ^Wall, Mike (September 12, 2013),"Voyager 1 Has Left Solar System",Web,Space.com,archivedfrom the original on 14 September 2013,retrieved13 September2013.
- ^Hughes, Amanda Jane; Soldini, Stefania (November 26, 2020),The solar discs that could power Earth,BBC,retrieved2024-05-29.
- ^Razani 2012,pp. 97–99.
- ^"Space Foundation Releases The Space Report 2023 Q2, Showing Annual Growth of Global Space Economy to $546B",Space Foundation,2023-07-25,retrieved2024-04-24.
- ^Bisset, Victoria (2023-02-04),"In a world of drones and satellites, why use a spy balloon anyway?",Washington Post,retrieved2024-04-24.
- ^Harrington, J. D.; et al. (12 December 2012),NASA's Hubble Provides First Census of Galaxies Near Cosmic Dawn,NASA, 12-428,archivedfrom the original on 22 March 2015.
- ^Landgraf, M.; et al. (February 2001), "IRSI/Darwin: peering through the interplanetary dust cloud",ESA Bulletin,105(105): 60–63,arXiv:astro-ph/0103288,Bibcode:2001ESABu.105...60L.
- ^Maccone, Claudio (August 2001), "Searching for bioastronomical signals from the farside of the Moon", in Ehrenfreund, P.; Angerer, O.; Battrick, B. (eds.),Exo-/astro-biology. Proceedings of the First European Workshop,vol. 496, Noordwijk: ESA Publications Division, pp. 277–280,Bibcode:2001ESASP.496..277M,ISBN978-92-9092-806-5.
- ^Chapmann, Glenn (May 22–27, 1991), "Space: the Ideal Place to Manufacture Microchips", in Blackledge, R.; Radfield, C.; Seida, S. (eds.),Proceedings of the 10th International Space Development Conference(PDF),San Antonio, Texas, pp. 25–33, archived fromthe original(PDF)on 2011-07-06,retrieved2010-01-12.
{{citation}}
:CS1 maint: location missing publisher (link) - ^Forgan, Duncan H.; Elvis, Martin (October 2011), "Extrasolar asteroid mining as forensic evidence for extraterrestrial intelligence",International Journal of Astrobiology,10(4): 307–313,arXiv:1103.5369,Bibcode:2011IJAsB..10..307F,doi:10.1017/S1473550411000127,S2CID119111392.
- ^Burton, Rodney; et al. (May 2005), "Low-Cost Launch of Payloads to Low Earth Orbit",Journal of Spacecraft and Rockets,43(3): 696–698,Bibcode:2006JSpRo..43..696B,doi:10.2514/1.16244.
- ^Bolonkin 2010,p. xv.
- ^Crawford, I. A. (September 1990), "Interstellar Travel: A Review for Astronomers",Quarterly Journal of the Royal Astronomical Society,31:377–400,Bibcode:1990QJRAS..31..377C.
- ^Yu, Xinxian; et al. (July 2022),"Enhanced radiative cooling paint with broken glass bubbles",Renewable Energy,194:129–136,doi:10.1016/j.renene.2022.05.094,S2CID248972097– via Elsevier Science Direct,
Radiative cooling does not consume external energy but rather harvests coldness from outer space as a new renewable energy source.
- ^Ma, Hongchen (2021),"Flexible Daytime Radiative Cooling Enhanced by Enabling Three-Phase Composites with Scattering Interfaces between Silica Microspheres and Hierarchical Porous Coatings",ACS Applied Materials & Interfaces,13(16): 19282–19290,arXiv:2103.03902,doi:10.1021/acsami.1c02145,PMID33866783,S2CID232147880– via ACS Publications,
Daytime radiative cooling has attracted considerable attention recently due to its tremendous potential for passively exploiting the coldness of the universe as clean and renewable energy.
- ^Zevenhovena, Ron; Fält, Martin (June 2018),"Radiative cooling through the atmospheric window: A third, less intrusive geoengineering approach",Energy,152:27,Bibcode:2018Ene...152...27Z,doi:10.1016/j.energy.2018.03.084– via Elsevier Science Direct,
An alternative, third geoengineering approach would be enhanced cooling by thermal radiation from the Earth's surface into space.
- ^Wang, Tong; et al. (2021), "A structural polymer for highly efficient all-day passive radiative cooling",Nature Communications,12(365): 365,doi:10.1038/s41467-020-20646-7,PMC7809060,PMID33446648,
One possibly alternative approach is passive radiative cooling—a sky-facing surface on the Earth spontaneously cools by radiating heat to the ultracold outer space through the atmosphere's longwave infrared (LWIR) transparency window (λ ~ 8–13 μm).
- ^Heo, Se-Yeon; et al. (June 2022),"Heat-shedding with photonic structures: radiative cooling and its potential",Journal of Materials Chemistry C,10(27): 9915–9937,doi:10.1039/D2TC00318J,S2CID249695930– via Royal Society of Chemistry.
Sources
- Barbieri, C. (2006),Fundamentals of Astronomy,CRC Press, p. 253,ISBN978-0-7503-0886-1
- Billings, Charles E. (1973), "Barometric Pressure", in Parker, James F.; West, Vita R. (eds.),Bioastronautics Data Book,vol. 3006 (2nd ed.),Bibcode:1973NASSP3006.....P,NASA SP-3006
- Bolonkin, Alexander (2010),Non-Rocket Space Launch and Flight,Elsevier,ISBN978-0-08-045875-5
- Borowitz, Sidney; Beiser, Arthur (1971),Essentials of physics: a text for students of science and engineering,Addison-Wesley series in physics (2nd ed.), Addison-Wesley Publishing CompanyNote: this source gives a value of2.7 × 1025molecules per cubic meter.
- Cajori, Florian (1917),A history of physics in its elementary branches: including the evolution of physical laboratories,New York: The Macmillan Company
- Chamberlain, Joseph Wyan (1978),Theory of planetary atmospheres: an introduction to their physics and chemistry,International geophysics series, vol. 22, Academic Press,ISBN978-0-12-167250-8
- Collins, Martin J. (2007),"Mariner 2 Mock-up",After Sputnik: 50 years of the Space Age,HarperCollins,ISBN978-0-06-089781-9
- Davies, P. C. W. (1977),The physics of time asymmetry,University of California Press,ISBN978-0-520-03247-7Note: a light year is about 1013km.
- Davis, Jeffrey R.; Johnson, Robert; Stepanek, Jan (2008),Fundamentals of Aerospace Medicine(4th ed.), Lippincott Williams & Wilkins,ISBN978-0-7817-7466-6
- Dickson, Paul (2010),A Dictionary of the Space Age,New Series in NASA History, JHU Press,ISBN978-0-8018-9504-3.
- Eckert, Michael (2006),The dawn of fluid dynamics: a discipline between science and technology,Wiley-VCH,ISBN978-3-527-40513-8
- Ellery, Alex (2000),An introduction to space robotics,Springer-Praxis books in astronomy and space sciences, Springer,ISBN978-1-85233-164-1
- Fichtner, Horst; Liu, W. William (2011), "Advances in Coordinated Sun-Earth System Science Through Interdisciplinary Initiatives and International Programs", written at Sopron, Hungary, in Miralles, M.P.; Almeida, J. Sánchez (eds.),The Sun, the Solar Wind, and the Heliosphere,IAGA Special Sopron Book Series, vol. 4, Berlin: Springer, pp. 341–345,Bibcode:2011sswh.book..341F,doi:10.1007/978-90-481-9787-3_24,ISBN978-90-481-9786-6
- Freedman, Roger A.; Kaufmann, William J. (2005),Universe(7th ed.), New York: W. H. Freeman and Company,ISBN978-0-7167-8694-8
- Frisch, Priscilla C.; Müller, Hans R.; Zank, Gary P.; Lopate, C. (May 6–9, 2002), "Galactic environment of the Sun and stars: interstellar and interplanetary material", in Livio, Mario; Reid, I. Neill; Sparks, William B. (eds.),Astrophysics of life. Proceedings of the Space Telescope Science Institute Symposium,Space Telescope Science Institute symposium series, vol. 16, Baltimore, MD, US: Cambridge University Press, p. 21,Bibcode:2005asli.symp...21F,ISBN978-0-521-82490-3
- Gatti, Hilary (2002),Giordano Bruno and Renaissance science,Cornell University Press,ISBN978-0-8014-8785-9
- Genz, Henning (2001),Nothingness: the science of empty space,Da Capo Press,ISBN978-0-7382-0610-3
- Ghosh, S. N. (2000),Atmospheric Science and Environment,Allied Publishers,ISBN978-81-7764-043-4
- Goodman, John M. (2006),Space Weather & Telecommunications,Springer Science & Business Media,ISBN978-0-387-23671-1
- Grant, Edward (1981),Much ado about nothing: theories of space and vacuum from the Middle Ages to the scientific revolution,The Cambridge history of science series, Cambridge University Press,ISBN978-0-521-22983-8
- Hardesty, Von; Eisman, Gene; Krushchev, Sergei (2008),Epic Rivalry: The Inside Story of the Soviet and American Space Race,National Geographic Books, pp. 89–90,ISBN978-1-4262-0321-3
- Hariharan, P. (2003),Optical interferometry(2nd ed.), Academic Press,ISBN978-0-12-311630-7
- Harris, Philip Robert (2008),Space enterprise: living and working offworld in the 21st century,Springer Praxis Books / Space Exploration Series, Springer,ISBN978-0-387-77639-2
- Harrison, Albert A. (2002),Spacefaring: The Human Dimension,University of California Press,ISBN978-0-520-23677-6
- Holton, Gerald James; Brush, Stephen G. (2001),"Physics, the human adventure: from Copernicus to Einstein and beyond",Physics Today,54(10) (3rd ed.), Rutgers University Press: 69,Bibcode:2001PhT....54j..69H,doi:10.1063/1.1420555,ISBN978-0-8135-2908-0
- Kanas, Nick; Manzey, Dietrich (2008), "Basic Issues of Human Adaptation to Space Flight",Space Psychology and Psychiatry,Space Technology Library, vol. 22, pp. 15–48,Bibcode:2008spp..book.....K,doi:10.1007/978-1-4020-6770-9_2,ISBN978-1-4020-6769-3.
- Kelly, Suzanne (1965),The de muno of William Gilbert,Amsterdam: Menno Hertzberger & Co.
- Koskinen, Hannu (2010),Physics of Space Storms: From the Surface of the Sun to the Earth,Environmental Sciences Series, Springer,ISBN978-3-642-00310-3
- Lang, Kenneth R. (1999),Astrophysical formulae: Radiation, gas processes, and high energy astrophysics,Astronomy and astrophysics library (3rd ed.), Birkhäuser,ISBN978-3-540-29692-8
- Liddle, Andrew (2015),An Introduction to Modern Cosmology,John Wiley,ISBN978-1-118-50214-3
- Lide, David R. (1993),CRC handbook of chemistry and physics(74th ed.), CRC Press,ISBN978-0-8493-0595-5
- Maor, Eli (1991),To infinity and beyond: a cultural history of the infinite,Princeton paperbacks,ISBN978-0-691-02511-7
- Mendillo, Michael (November 8–10, 2000), "The atmosphere of the moon", in Barbieri, Cesare; Rampazzi, Francesca (eds.),Earth-Moon Relationships,Padova, Italy at the Accademia Galileiana Di Scienze Lettere Ed Arti: Springer, p. 275,ISBN978-0-7923-7089-5
- Needham, Joseph; Ronan, Colin (1985),The Shorter Science and Civilisation in China,vol. 2, Cambridge University Press,ISBN978-0-521-31536-4
- O'Leary, Beth Laura (2009), Darrin, Ann Garrison (ed.),Handbook of space engineering, archaeology, and heritage,Advances in engineering, CRC Press,ISBN978-1-4200-8431-3
- Olenick, Richard P.; Apostol, Tom M.; Goodstein, David L. (1986),Beyond the mechanical universe: from electricity to modern physics,Cambridge University Press,ISBN978-0-521-30430-6
- Orloff, Richard W. (2001),Apollo by the Numbers: A Statistical Reference,NASA,ISBN978-0-16-050631-4,retrieved2008-01-28
- Papagiannis, Michael D. (1972),Space Physics and Space Astronomy,Taylor & Francis,ISBN978-0-677-04000-4
- Piantadosi, Claude A. (2003),The Biology of Human Survival: Life and Death in Extreme Environments,Oxford University Press,ISBN978-0-19-974807-5
- Porter, Roy; Park, Katharine; Daston, Lorraine (2006), "The Cambridge History of Science: Early modern science",Early Modern Science,vol. 3, Cambridge University Press, p. 27,ISBN978-0-521-57244-6
- Prialnik, Dina (2000),An Introduction to the Theory of Stellar Structure and Evolution,Cambridge University Press,ISBN978-0-521-65937-6,retrieved2015-03-26
- Rauchfuss, Horst (2008),Chemical Evolution and the Origin of Life,Translated by T. N. Mitchell, Springer,ISBN978-3-540-78822-5
- Razani, Mohammad (2012),Information Communication and Space Technology,CRC Press,ISBN978-1-4398-4163-1
- Schrijver, Carolus J.;Siscoe, George L.(2010),Heliophysics: Evolving Solar Activity and the Climates of Space and Earth,Cambridge University Press,ISBN978-0-521-11294-9
- Silk, Joseph(2000),The Big Bang(3rd ed.), Macmillan,ISBN978-0-8050-7256-3
- Sparke, Linda S.;Gallagher, John S. (2007),Galaxies in the Universe: An Introduction(2nd ed.), Cambridge University Press,Bibcode:2007gitu.book.....S,ISBN978-0-521-85593-8
- Spitzer, Lyman Jr. (1978),Physical Processes in the Interstellar Medium,Wiley Classics Library,ISBN978-0-471-29335-4
- Stuart Wortley, Emmeline Charlotte E.(1841),The maiden of Moscow, a poem,How and Parsons, Canto X, section XIV, lines 14–15,
All Earth in madness moved,—o'erthrown, / To outer space—driven—racked—undone!
- Tassoul, Jean Louis; Tassoul, Monique (2004),A concise history of solar and stellar physics,Princeton University Press,ISBN978-0-691-11711-9
- Thagard, Paul (1992),Conceptual revolutions,Princeton University Press,ISBN978-0-691-02490-5
- Tyson, Neil deGrasse;Goldsmith, Donald (2004),Origins: fourteen billion years of cosmic evolution,W. W. Norton & Company, pp. 114–115,ISBN978-0-393-05992-2
- United States (2016),United States Code 2006 Edition Supplement V,Washington D. C.: United States Government Printing Office, p. 536
- Von Humboldt, Alexander(1845),Cosmos: a survey of the general physical history of the Universe,New York: Harper & Brothers Publishers,hdl:2027/nyp.33433071596906
- Webb, Stephen (1999),Measuring the universe: the cosmological distance ladder,Springer,ISBN978-1-85233-106-1
- Williamson, Mark (2006),Spacecraft Technology: The Early Years,History and Management of Technology Series, vol. 33, IET,ISBN978-0-86341-553-1
- Wong, Wilson; Fergusson, James Gordon (2010),Military space power: a guide to the issues,Contemporary military, strategic, and security issues, ABC-CLIO,ISBN978-0-313-35680-3
- Wszolek, Bogdan (2013),"Is there Matter in Voids?",in Arp, H. C.; Keys, C. R.; Rudnicki, K. (eds.),Progress in New Cosmologies: Beyond the Big Bang,Springer Science & Business Media,ISBN978-1-4899-1225-1