Abstract
Interacting mixtures of bosons and fermions are ubiquitous in nature. They form the backbone of the standard model of physics, provide a framework for understanding quantum materials and are of technological importance in helium dilution refrigerators. However, the description of their coupled thermodynamics and collective behaviour is challenging. Bose–Fermi mixtures of ultracold atoms provide a platform to investigate their properties in a highly controllable environment, where the species concentration and interaction strength can be tuned at will. Here we characterize the collective oscillations of spin-polarized fermionic impurities immersed in a Bose–Einstein condensate as a function of the interaction strength and temperature. For strong interactions, the Fermi gas perfectly mimics the superfluid hydrodynamic modes of the condensate, from low-energy quadrupole modes to high-order Faraday excitations. With an increasing number of bosonic thermal excitations, the dynamics of the impurities cross over from the collisionless to the hydrodynamic regime, reminiscent of the emergence of hydrodynamics in two-dimensional electron fluids.
This is a preview of subscription content,access via your institution
Access options
Access Nature and 54 other Nature Portfolio journals
Get Nature+, our best-value online-access subscription
24,99 €/ 30 days
cancel any time
Subscribe to this journal
Receive 12 print issues and online access
251,40 € per year
only 20,95 € per issue
Buy this article
- Purchase on SpringerLink
- Instant access to full article PDF
Prices may be subject to local taxes which are calculated during checkout
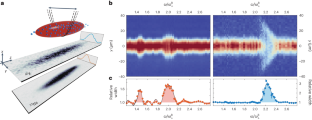
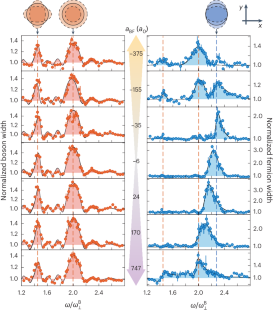
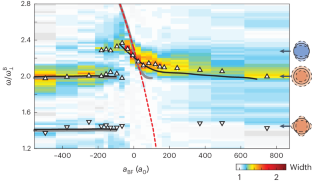
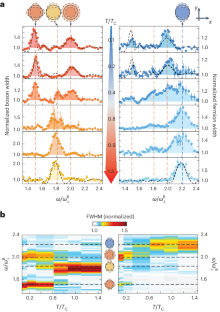

Similar content being viewed by others
Data availability
The data that support the plots within this paper and other findings of this study are available as source data files. All other data are available from the corresponding author upon reasonable request.
References
Landau, L. D. The movement of electrons in the crystal lattice.Phys. Z. Sowjetunion3,644 (1933).
Pekar, S. I. Autolocalization of the electron in an inertially polarizable dielectric medium.Zh. Eksp. Teor. Fiz.16,335 (1946).
Ebner, C. & Edwards, D. The low temperature thermodynamic properties of superfluid solutions of3He in4He.Phys. Rep.2,77–154 (1971).
Schaefer, B.-J. & Wambach, J. The phase diagram of the quark–meson model.Nucl. Phys. A757,479–492 (2005).
Sidler, M. et al. Fermi polaron-polaritons in charge-tunable atomically thin semiconductors.Nat. Phys.13,255–261 (2017).
Shimazaki, Y. et al. Strongly correlated electrons and hybrid excitons in a moiréheterostructure.Nature580,472–477 (2020).
Schwartz, I. et al. Electrically tunable Feshbach resonances in twisted bilayer semiconductors.Science374,336–340 (2021).
Hadzibabic, Z. et al. Two-species mixture of quantum degenerate Bose and Fermi gases.Phys. Rev. Lett.88,160401 (2002).
Stan, C. A., Zwierlein, M. W., Schunck, C. H., Raupach, S. M. F. & Ketterle, W. Observation of Feshbach resonances between two different atomic species.Phys. Rev. Lett.93,143001 (2004).
Inouye, S. et al. Observation of heteronuclear Feshbach resonances in a mixture of bosons and fermions.Phys. Rev. Lett.93,183201 (2004).
Silber, C. et al. Quantum-degenerate mixture of fermionic lithium and bosonic rubidium gases.Phys. Rev. Lett.95,170408 (2005).
Ospelkaus, S., Ospelkaus, C., Humbert, L., Sengstock, K. & Bongs, K. Tuning of heteronuclear interactions in a degenerate Fermi–Bose mixture.Phys. Rev. Lett.97,120403 (2006).
Zaccanti, M. et al. Control of the interaction in a Fermi–Bose mixture.Phys. Rev. A74,041605 (2006).
Shin, Y.-i, Schirotzek, A., Schunck, C. H. & Ketterle, W. Realization of a strongly interacting Bose–Fermi mixture from a two-component Fermi gas.Phys. Rev. Lett.101,070404 (2008).
Wu, C.-H., Santiago, I., Park, J. W., Ahmadi, P. & Zwierlein, M. W. Strongly interacting isotopic Bose–Fermi mixture immersed in a Fermi sea.Phys. Rev. A84,011601 (2011).
Park, J. W. et al. Quantum degenerate Bose–Fermi mixture of chemically different atomic species with widely tunable interactions.Phys. Rev. A85,051602 (2012).
Vaidya, V. D., Tiamsuphat, J., Rolston, S. L. & Porto, J. V. Degenerate Bose–Fermi mixtures of rubidium and ytterbium.Phys. Rev. A92,043604 (2015).
Trautmann, A. et al. Dipolar quantum mixtures of erbium and dysprosium atoms.Phys. Rev. Lett.121,213601 (2018).
Lous, R. S. et al. Probing the interface of a phase-separated state in a repulsive Bose–Fermi mixture.Phys. Rev. Lett.120,243403 (2018).
DeSalvo, B. J., Patel, K., Cai, G. & Chin, C. Observation of fermion-mediated interactions between bosonic atoms.Nature568,61–64 (2019).
Patel, K., Cai, G., Ando, H. & Chin, C. Sound propagation in a Bose–Fermi mixture: from weak to strong interactions.Phys. Rev. Lett.131,083003 (2023).
Viverit, L., Pethick, C. J. & Smith, H. Zero-temperature phase diagram of binary boson–fermion mixtures.Phys. Rev. A61,053605 (2000).
Büchler, H. P. & Blatter, G. Supersolid versus phase separation in atomic Bose–Fermi mixtures.Phys. Rev. Lett.91,130404 (2003).
Bertaina, G., Fratini, E., Giorgini, S. & Pieri, P. Quantum Monte Carlo study of a resonant Bose–Fermi mixture.Phys. Rev. Lett.110,115303 (2013).
Kinnunen, J. J. & Bruun, G. M. Induced interactions in a superfluid Bose–Fermi mixture.Phys. Rev. A91,041605 (2015).
Ludwig, D., Floerchinger, S., Moroz, S. & Wetterich, C. Quantum phase transition in Bose-Fermi mixtures.Phys. Rev. A84,033629 (2011).
Ferrier-Barbut, I. et al. A mixture of Bose and Fermi superfluids.Science345,1035–1038 (2014).
Delehaye, M. et al. Critical velocity and dissipation of an ultracold Bose–Fermi counterflow.Phys. Rev. Lett.115,265303 (2015).
Yao, X.-C. et al. Observation of coupled vortex lattices in a mass-imbalance Bose and Fermi superfluid mixture.Phys. Rev. Lett.117,145301 (2016).
Roy, R., Green, A., Bowler, R. & Gupta, S. Two-element mixture of Bose and Fermi superfluids.Phys. Rev. Lett.118,055301 (2017).
Wu, Y. P. et al. Coupled dipole oscillations of a mass-imbalanced Bose–Fermi superfluid mixture.Phys. Rev. B97,020506 (2018).
Ospelkaus, C., Ospelkaus, S., Sengstock, K. & Bongs, K. Interaction-driven dynamics of40K–87Rb fermion–boson gas mixtures in the large-particle-number limit.Phys. Rev. Lett.96,020401 (2006).
Huang, B. et al. Breathing mode of a Bose–Einstein condensate repulsively interacting with a fermionic reservoir.Phys. Rev. A99,041602 (2019).
Hu, M.-G. et al. Bose polarons in the strongly interacting regime.Phys. Rev. Lett.117,055301 (2016).
Yan, Z. Z., Ni, Y., Robens, C. & Zwierlein, M. W. Bose polarons near quantum criticality.Science368,190–194 (2020).
Landau, L. D. Two-fluid model of liquid helium II.J. Phys. USSR5,71–90 (1941).
Chevy, F. Counterflow in a doubly superfluid mixture of bosons and fermions.Phys. Rev. A91,063606 (2015).
Seetharam, K., Shchadilova, Y., Grusdt, F., Zvonarev, M. B. & Demler, E. Dynamical quantum Cherenkov transition of fast impurities in quantum liquids.Phys. Rev. Lett.127,185302 (2021).
Seetharam, K., Shchadilova, Y., Grusdt, F., Zvonarev, M. & Demler, E. Quantum Cherenkov transition of finite momentum Bose polarons. Preprint athttps://arxiv.org/abs/2109.12260(2021).
Miyakawa, T., Suzuki, T. & Yabu, H. Sum-rule approach to collective oscillations of a boson–fermion mixed condensate of alkali-metal atoms.Phys. Rev. A62,063613–063611 (2000).
Yip, S. K. Collective modes in a dilute Bose–Fermi mixture.Phys. Rev. A64,023609 (2001).
Sogo, T., Miyakawa, T., Suzuki, T. & Yabu, H. Random-phase approximation study of collective excitations in the Bose–Fermi mixed condensate of alkali-metal gases.Phys. Rev. A66,136181–1361812 (2002).
Liu, X. J. & Hu, H. Collisionless and hydrodynamic excitations of trapped boson–fermion mixtures.Phys. Rev. A67,023613 (2003).
Capuzzi, P., Minguzzi, A. & Tosi, M. P. Collisional oscillations of trapped boson–fermion mixtures in the approach to the collapse instability.Phys. Rev. A69,053615 (2004).
Imambekov, A. & Demler, E. Exactly solvable case of a one-dimensional Bose–Fermi mixture.Phys. Rev. A73,021602 (2006).
Banerjee, A. Dipole oscillations of a Bose–Fermi mixture: effect of unequal masses of Bose and Fermi particles.J. Phys. B42,235301 (2009).
Van Schaeybroeck, B. & Lazarides, A. Trapped phase-segregated Bose–Fermi mixtures and their collective excitations.Phys. Rev. A79,033618 (2009).
Maruyama, T., Yamamoto, T., Nishimura, T. & Yabu, H. Deformation dependence of breathing oscillations in Bose–Fermi mixtures at zero temperature.J. Phys. B47,25–34 (2014).
Asano, Y., Narushima, M., Watabe, S. & Nikuni, T. Collective excitations in Bose–Fermi mixtures.J. Low Temp. Phys.196,133–139 (2019).
Ono, Y., Hatsuda, R., Shiina, K., Mori, H. & Arahata, E. Three sound modes in a Bose–Fermi superfluid mixture at finite temperatures.J. Phys. Soc. Jpn88,034003 (2019).
Zhang, J. et al. Anomalous thermal diffusivity in underdoped YBa2Cu3O6+x.Proc. Natl Acad. Sci. USA114,5378–5383 (2017).
Gooth, J. et al. Thermal and electrical signatures of a hydrodynamic electron fluid in tungsten diphosphide.Nat. Commun.9,4093 (2018).
Jin, D. S., Ensher, J. R., Matthews, M. R., Wieman, C. E. & Cornell, E. A. Collective excitations of a Bose–Einstein condensate in a dilute gas.Phys. Rev. Lett.77,420–423 (1996).
Mewes, M. O. et al. Collective excitations of a Bose–Einstein condensate in a magnetic trap.Phys. Rev. Lett.77,988–991 (1996).
Stamper-Kurn, D. M., Miesner, H. J., Inouye, S., Andrews, M. R. & Ketterle, W. Collisionless and hydrodynamic excitations of a Bose–Einstein condensate.Phys. Rev. Lett.81,500–503 (1998).
Gensemer, S. D. & Jin, D. S. Transition from collisionless to hydrodynamic behavior in an ultracold Fermi gas.Phys. Rev. Lett.87,173201 (2001).
O’Hara, K. M., Hemmer, S. L., Gehm, M. E., Granade, S. R. & Thomas, J. E. Observation of a strongly interacting degenerate Fermi gas of atoms.Science298,2179–2182 (2002).
Regal, C. A. & Jin, D. S. Measurement of positive and negative scattering lengths in a Fermi gas of atoms.Phys. Rev. Lett.90,230404 (2003).
Bourdel, T. et al. Measurement of the interaction energy near a Feshbach resonance in a6Li Fermi gas.Phys. Rev. Lett.91,020402 (2003).
Trenkwalder, A. et al. Hydrodynamic expansion of a strongly interacting Fermi–Fermi mixture.Phys. Rev. Lett.106,115304 (2011).
Tey, M. K. et al. Collective modes in a unitary Fermi gas across the superfluid phase transition.Phys. Rev. Lett.110,055303 (2013).
Ravensbergen, C. et al. Resonantly interacting Fermi–Fermi mixture of161Dy and40K.Phys. Rev. Lett.124,203402 (2020).
Ferlaino, F. et al. Dipolar oscillations in a quantum degenerate Fermi–Bose atomic mixture.J. Opt. B5,S3–S8 (2003).
Fukuhara, T., Tsujimoto, T. & Takahashi, Y. Quadrupole oscillations in a quantum degenerate Bose–Fermi mixture.Appl. Phys. B96,271–274 (2009).
Kagan, M. Y. & Bianconi, A. Fermi–Bose mixtures and BCS–BEC crossover in high-Tcsuperconductors.Condens. Matter4,51 (2019).
Stringari, S. Collective excitations of a trapped Bose-condensed gas.Phys. Rev. Lett.77,2360–2363 (1996).
Pethick, C. & Smith, H.Bose–Einstein Condensation in Dilute Gases(Cambridge Univ. Press, 2008).
Castin, Y. & Dum, R. Bose–Einstein condensates in time dependent traps.Phys. Rev. Lett.77,5315–5319 (1996).
DeMarco, B., Bohn, J. L., Burke, J. P., Holland, M. & Jin, D. S. Measurement of p-wave threshold law using evaporatively cooled fermionic atoms.Phys. Rev. Lett.82,4208–4211 (1999).
Dolgirev, P. E. et al. Accelerating analysis of Boltzmann equations using Gaussian mixture models: application to quantum Bose–Fermi mixtures. Preprint athttps://arxiv.org/abs/2304.09911(2022).
Menotti, C., Pedri, P. & Stringari, S. Expansion of an interacting Fermi gas.Phys. Rev. Lett.89,250402 (2002).
Faraday, M. On a peculiar class of acoustical figures; and on certain forms assumed by groups of particles upon vibrating elastic surfaces.Phil. Trans. R. Soc. Lond.121,299–340 (1831).
Engels, P., Atherton, C. & Hoefer, M. A. Observation of Faraday waves in a Bose–Einstein condensate.Phys. Rev. Lett.98,095301 (2007).
Groot, A.Excitations in Hydrodynamic Ultra-Cold Bose Gases.PhD thesis, Utrecht Univ. (2015).
Nguyen, J. H. et al. Parametric excitation of a Bose–Einstein condensate: from Faraday waves to granulation.Phys. Rev. X9,11052 (2019).
Heiselberg, H., Pethick, C. J., Smith, H. & Viverit, L. Influence of induced interactions on the superfluid transition in dilute Fermi gases.Phys. Rev. Lett.85,2418–2421 (2000).
Bijlsma, M. J., Heringa, B. A. & Stoof, H. T. Phonon exchange in dilute Fermi–Bose mixtures: tailoring the Fermi–Fermi interaction.Phys. Rev. A61,053601 (2000).
Efremov, D. V. & Viverit, L. p-wave Cooper pairing of fermions in mixtures of dilute Fermi and Bose gases.Phys. Rev. B65,134519 (2002).
Matera, F. Fermion pairing in Bose–Fermi mixtures.Phys. Rev. A68,043624 (2003).
Kinnunen, J. J., Wu, Z. & Bruun, G. M. Induced p-wave pairing in Bose-Fermi mixtures.Phys. Rev. Lett.121,253402 (2018).
Acknowledgements
We acknowledge E. Wolf for helpful discussions. We acknowledge support from NSF, AFOSR through a MURI on Ultracold Molecules, the Vannevar Bush Faculty Fellowship. Z.Z.Y. and A.C. acknowledge support from the NSF GRFP. C.R. acknowledges support from the Deutsche Forschungsgemeinschaft (DFG) Germany research fellowship (421987027). K.S. acknowledges funding from NSF EAGER-QAC-QCH award no. 2037687. P.D. and E.D. were supported by ARO grant number W911NF-20-1-0163, SNSF project 200021-212899. E.D. acknowledges support from the Swiss National Science Foundation under Division II.
Author information
Authors and Affiliations
Contributions
M.Z. and E.D. conceived of the experiments and supervised the study. Z.Z.Y., Y.N., A.C. and C.R. performed the experiments and the data analysis. Z.Z.Y., C.R. and M.Z. performed the numerical calculations for the Boltzmann equations without collisions and the mean-field scaling ansatz. P.E.D., K.S. and E.D. performed the theoretical and numerical calculations on the high-temperature Boltzmann equations. All authors contributed to the paper and the interpretation of data.
Corresponding author
Ethics declarations
Competing interests
The authors declare no competing interests.
Peer review
Peer review information
Nature Physicsthanks Xing-Can Yao and the other, anonymous, reviewer(s) for their contribution to the peer review of this work.
Additional information
Publisher’s noteSpringer Nature remains neutral with regard to jurisdictional claims in published maps and institutional affiliations.
Supplementary information
Supplementary Information
Supplementary Figs. 1–8 and Discussion.
Source data
Source Data Fig. 1
Widths versus frequency for non-interacting Bose–Fermi mixture.
Source Data Fig. 2
Widths versus frequency for Bose–Fermi mixture across different interaction strengths, and their error bars.
Source Data Fig. 3
Files for the colour plots of the fermionic response versus frequency and scattering length, and the theoretical curves as described in the figure caption.
Source Data Fig. 4
Bose and Fermi widths versus frequency.
Source Data Fig. 5
Data for the Faraday mode plots.
Rights and permissions
Springer Nature or its licensor (e.g. a society or other partner) holds exclusive rights to this article under a publishing agreement with the author(s) or other rightsholder(s); author self-archiving of the accepted manuscript version of this article is solely governed by the terms of such publishing agreement and applicable law.
About this article
Cite this article
Yan, Z.Z., Ni, Y., Chuang, A.et al.Collective flow of fermionic impurities immersed in a Bose–Einstein condensate. Nat. Phys.20,1395–1400 (2024). https://doi.org/10.1038/s41567-024-02541-w
Received:
Accepted:
Published:
Issue Date:
DOI:https://doi.org/10.1038/s41567-024-02541-w