Abstract
Dispersants are globally and routinely applied as an emergency response to oil spills in marine ecosystems with the goal of chemically enhancing the dissolution of oil into water, which is assumed to stimulate microbially mediated oil biodegradation. However, little is known about how dispersants affect the composition of microbial communities or their biodegradation activities. The published findings are controversial, probably owing to variations in laboratory methods, the selected model organisms and the chemistry of different dispersant–oil mixtures. Here, we argue that an in-depth assessment of the impacts of dispersants on microorganisms is needed to evaluate the planning and use of dispersants during future responses to oil spills.
This is a preview of subscription content, access via your institution
Access options
Subscribe to this journal
Receive 12 print issues and online access
195,33 € per year
only 16,28 € per issue
Buy this article
- Purchase on SpringerLink
- Instant access to full article PDF
Prices may be subject to local taxes which are calculated during checkout
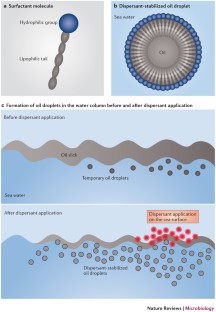
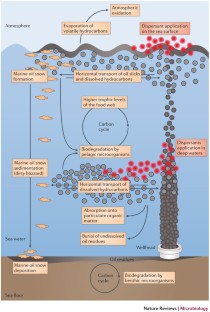
Similar content being viewed by others
References
Leahy, J. G. & Colwell, R. R. Microbial degradation of hydrocarbons in the environment. Microbiol. Rev. 54, 305–315 (1990).
Tissot, B. P. & Welte, D. H. Petroleum Formation and Occurrence: A New Approach to Oil and Gas Exploration 2nd edn (Springer, 1984).
Putscher, R. E. Isolation of olefins from Bradford crude oil. Anal. Chem. 24, 1551–1558 (1952).
Kvenvolden, K. A. & Cooper, C. K. Natural seepage of crude oil into the marine environment. Geo-Mar. Lett. 23, 140–146 (2003).
National Research Council. Oil in the Sea III: Inputs, Fates, and Effects (National Academies Press, 2003).
Joye, S. B., Bowles, M. W., Samarkin, V. A., Hunter, K. S. & Niemann, H. Biogeochemical signatures and microbial activity of different cold-seep habitats along the Gulf of Mexico deep slope. Deep-Sea Res. II 57, 1990–2001 (2010).
Hazen, T. C. et al. Deep-sea oil plume enriches indigenous oil-degrading bacteria. Science 330, 204–208 (2010).
Ziervogel, K. et al. Microbial activities and dissolved organic matter dynamics in oil-contaminated surface seawater from the Deepwater Horizon oil spill site. PLoS ONE 7, e34816 (2012).
Desai, J. D. & Banat, I. M. Microbial production of surfactants and their commercial potential. Microbiol. Mol. Biol. Rev. 61, 47–64 (1997).
Passow, U. Formation of rapidly-sinking, oil-associated marine snow. Deep-Sea Res. II http://dx.doi.org/10.1016/j.dsr2.2014.10.001 (2014).
Joye, S. B., Teske, A. P. & Kostka, J. E. Microbial dynamics following the Macondo oil well blowout across Gulf of Mexico environments. BioScience 64, 766–777 (2014).
Steen, A. Frequency of dispersant use worldwide. Int. Oil Spill Con. Proc. 2008, 645–650 (2008).
Bruheim, P., Bredholt, H. & Eimhjellen, K. Effects of surfactant mixtures, including Corexit 9527, on bacterial oxidation of acetate and alkanes in crude oil. Appl. Environ. Microbiol. 65, 1658–1661 (1999).
Foght, J. M. & Westlake, D. W. S. Effect of the dispersant Corexit 9527 on the microbial degradation of Prudhoe Bay oil. Can. J. Microbiol. 28, 117–122 (1982).
Macias-Zamora, J. V., Meléndez-Sánchez, A. L., Ramírez-Álvarez, N., Gutiérrez-Galindo, E. A. & Orozco-Borbón, M. V. On the effects of the dispersant Corexit 9500© during the degradation process of n-alkanes and PAHs in marine sediments. Environ. Monit. Assess. 186, 1051–1061 (2014).
Bælum, J. et al. Deep-sea bacteria enriched by oil and dispersant from the Deepwater Horizon spill. Environ. Microbiol. 14, 2405–2416 (2012).
Lindstrom, J. E. & Braddock, J. F. Biodegradation of petroleum hydrocarbons at low temperature in the presence of the dispersant Corexit 9500. Mar. Pollut. Bull. 44, 739–747 (2002).
Chakraborty, R., Borglin, S. E., Dubinsky, E. A., Andersen, G. L. & Hazen, T. C. Microbial response to the MC-252 oil and corexit 9500 in the Gulf of Mexico. Front. Microbiol. 3, 357 (2012).
Chandrasekar, S., Sorial, G. A. & Weaver, J. W. Dispersant effectiveness on oil spills — impact of salinity. ICES J. Mar. Sci. 63, 1418–1430 (2006).
Fingas, M., Wang, Z., Fieldhouse, B. & Smith, P. Chemical characteristics of an oil and the relationship to dispersant effectiveness. BSEE [online], (2003).
Weaver, J. W. Characteristics of spilled oils, fuels and petroleum products: 3a. Simulation of oil spills and dispersants under conditions of uncertainty. EPA [online], (2004).
Kuhl, A. J., Nyman, J. A., Kaller, M. D. & Green, C. C. Dispersant and salinity effects on weathering and acute toxicity of South Louisiana crude oil. Environ. Toxicol. Chem. 32, 2611–2620 (2013).
Jernelöv, A. & Olof, L. Ixtoc I: a case study of the world's largest oil spill. Ambio 10, 299–306 (1981).
Lewis, A., Crosbie, A., Davies, L. & Lunel, T. Dispersion of Emulsified Oils at Sea (AEA Technology, 1998).
Gilfillan, E. S. et al. Tidal area dispersant experiment, Searsport Maine: an overview. Int. Oil Spill Con. Proc. 1985, 553–559 (1985).
Prince, R. C. et al. The primary biodegradation of dispersed crude oil in the sea. Chemosphere 90, 521–526 (2013).
Moore, C. M. et al. Processes and patterns of oceanic nutrient limitation. Nature Geosci. 6, 701–710 (2013).
Edwards, B. R. et al. Rapid microbial respiration of oil from the Deepwater Horizon spill in offshore surface waters of the Gulf of Mexico. Environ. Res. Lett. 6, 035301 (2011).
Yoshida, A. et al. Microbial responses using denaturing gradient gel electrophoresis to oil and chemical dispersant in enclosed ecosystems. Mar. Pollut. Bull. 52, 89–95 (2006).
Martha, D. & Mulligan, C. N. Rhamnolipid biosurfactant assisted dispersion and biodegradation of spilled oil on surface waters. Proc. Annu. Conf. Can. Soc. Civil Engineer (2005).
Zahed, M., Aziz, H., Isa, M. & Mohajeri, L. Effect of initial oil concentration and dispersant on crude oil biodegradation in contaminated seawater. Bull. Environ. Contam. Toxicol. 84, 438–442 (2010).
Mason, O. U. et al. Metagenomics reveals sediment microbial community response to Deepwater Horizon oil spill. ISME J. 8, 1464–1475 (2014).
Committee on Understanding Oil Spill Dispersants. Oil Spill Dispersants: Efficacy and Effects (National Academies Press, 2005).
Pham, P. H., Huang, Y. J., Chen, C. & Bols, N. C. Corexit 9500 inactivates two enveloped viruses of aquatic animals but enhances the infectivity of a nonenveloped fish virus. Appl. Environ. Microbiol. 80, 1035–1041 (2014).
Fuller, C. et al. Comparative toxicity of oil, dispersant, and oil plus dispersant to several marine species. Environ. Toxicol. Chem. 23, 2941–2949 (2004).
Bulich, A. A. & Isenberg, D. L. Use of the luminescent bacterial system for the rapid assessment of aquatic toxicity. ISA Trans. 20, 29–33 (1981).
Fernández, A., Tejedor, C., Cabrera, F. & Chordi, A. Assessment of toxicity of river water and effluents by the bioluminescence assay using Photobacterium phosphoreum. Water Res. 29, 1281–1286 (1995).
Guzzella, L. & Mingazzini, M. Biological assaying of organic compounds in surface waters. Water Sci. Technol. 30, 113–124 (1994).
Hao, O. J., Shin, C.-J., Lin, C.-F., Jeng, F.-T. & Chen, Z.-C. Use of microtox tests for screening industrial wastewater toxicity. Water Sci. Technol. 34, 43–50 (1996).
Gustavson, K. E., Svenson, A. & Harkin, J. M. Comparison of toxicities and mechanism of action of n-alkanols in the submitochondrial particle and the Vibrio fischeri bioluminescence (Microtox®) bioassay. Environ. Toxicol. Chem. 17, 1917–1921 (1998).
Johnson, B. T. & Long, E. R. Rapid toxicity assessment of sediments from estuarine ecosystems: a new tandem in vitro testing approach. Environ. Toxicol. Chem. 17, 1099–1106 (1998).
Svenson, A., Edsholt, E., Ricking, M., Remberger, M. & Röttorp, J. Sediment contaminants and microtox toxicity tested in a direct contact exposure test. Environ. Toxicol. Water Qual. 11, 293–300 (1996).
Gälli, R., Munz, C. D. & Scholtz, R. Evaluation and application of aquatic toxicity tests: use of the Microtox test for the prediction of toxicity based upon concentrations of contaminants in soil. Hydrobiologia 273, 179–189 (1994).
Radniecki, T. S., Schneider, M. C. & Semprini, L. The influence of Corexit 9500A and weathering on Alaska North Slope crude oil toxicity to the ammonia oxidizing bacterium, Nitrosomonas europaea. Mar. Pollut. Bull. 68, 64–70 (2013).
Nelson-Smith, A. Oil Pollution and Marine Ecology (Plenum Press, 1973).
Nagell, B., Notini, M. & Grahn, O. Toxicity of four oil dispersants to some animals from the Baltic Sea. Mar. Biol. 28, 237–243 (1974).
Hamdan, L. & Fulmer, P. Effects of COREXIT®EC9500A on bacteria from a beach oiled by the Deepwater Horizon spill. Aquat. Microb. Ecol. 63, 101–109 (2011).
Ortmann, A. C. et al. Dispersed oil disrupts microbial pathways in pelagic food webs. PLoS ONE 7, e42548 (2012).
Zuijdgeest, A. & Huettel, M. Dispersants as used in response to the MC252-spill lead to higher mobility of polycyclic aromatic hydrocarbons in oil-contaminated Gulf of Mexico sand. PLoS ONE 7, e50549 (2012).
Johnson, B. T. in Small-scale Freshwater Toxicity Investigations (eds Blaise, C. & Férard, J.-F.) 69–105 (Springer, 2005).
Rosen, G., Osorio-Robayo, A., Rivera-Duarte, I. & Lapota, D. Comparison of bioluminescent Dinoflagellate (QwikLite) and bacterial (Microtox) rapid bioassays for the detection of metal and ammonia toxicity. Arch. Environ. Contam. Toxicol. 54, 606–611 (2008).
Paul, J. H. et al. Toxicity and mutagenicity of Gulf of Mexico waters during and after the Deepwater Horizon oil spill. Environ. Sci. Technol. 47, 9651–9659 (2013).
US Environmental Protection Agency. Alphabetical list of NCP product schedule (products available for use during an oil spill). EPA [online], (2013).
Singer, M. M. et al. Standardization of the preparation and quantitation of water-accommodated fractions of petroleum for toxicity testing. Mar. Pollut. Bull. 40, 1007–1016 (2000).
White, H. K. et al. Long-term persistence of dispersants following the Deepwater Horizon oil spill. Environ. Sci. Technol. Lett. 1, 295–299 (2014).
Venkataraman, P. et al. Attachment of a hydrophobically modified biopolymer at the oil-water interface in the treatment of oil spills. ACS Appl. Mater. Interfaces 5, 3572–3580 (2013).
Yakimov, M. M. et al. Alcanivorax borkumensis gen. nov., sp. nov., a new, hydrocarbon-degrading and surfactant-producing marine bacterium. Int. J. Syst. Bacteriol. 48, 339–348 (1998).
Dyksterhouse, S. E., Gray, J. P., Herwig, R. P., Lara, J. C. & Staley, J. T. Cycloclasticus pugetii gen. nov., sp. nov., an aromatic hydrocarbon-degrading bacterium from marine sediments. Int. J. Syst. Bacteriol. 45, 116–123 (1995).
Golyshin, P. N. et al. Oleiphilaceae fam. nov., to include Oleiphilus messinensis gen. nov., sp. nov., a novel marine bacterium that obligately utilizes hydrocarbons. Int. J. Syst. Evol. Microbiol. 52, 901–911 (2002).
Yakimov, M. M. et al. Oleispira antarctica gen. nov., sp. nov., a novel hydrocarbonoclastic marine bacterium isolated from Antarctic coastal sea water. Int. J. Syst. Evol. Microbiol. 53, 779–785 (2003).
Yakimov, M. M. et al. Thalassolituus oleivorans gen. nov., sp. nov., a novel marine bacterium that obligately utilizes hydrocarbons. Int. J. Syst. Evol. Microbiol. 54, 141–148 (2004).
Engelhardt, M. A., Daly, K., Swannell, R. P. & Head, I. M. Isolation and characterization of a novel hydrocarbon-degrading, Gram-positive bacterium, isolated from intertidal beach sediment, and description of Planococcus alkanoclasticus sp. nov. J. Appl. Microbiol. 90, 237–247 (2001).
Kleindienst, S. et al. Diverse sulfate-reducing bacteria of the Desulfosarcina/Desulfococcus clade are the key alkane degraders at marine seeps. ISME J. 8, 2029–2044 (2014).
Kleindienst, S., Ramette, A., Amann, R. & Knittel, K. Distribution and in situ abundance of sulfate-reducing bacteria in diverse marine hydrocarbon seep sediments. Environ. Microbiol. 14, 2689–2710 (2012).
Orcutt, B. N. et al. Impact of natural oil and higher hydrocarbons on microbial diversity, distribution, and activity in Gulf of Mexico cold-seep sediments. Deep-Sea Res. II 57, 2008–2021 (2010).
Shao, Z. & Wang, W. Enzymes and genes involved in aerobic alkane degradation. Front. Microbiol. 4, 116 (2013).
Sabirova, J. S., Ferrer, M., Regenhardt, D., Timmis, K. N. & Golyshin, P. N. Proteomic insights into metabolic adaptations in Alcanivorax borkumensis induced by alkane utilization. J. Bacteriol. 188, 3763–3773 (2006).
Kessler, J. D. et al. A persistent oxygen anomaly reveals the fate of spilled methane in the deep Gulf of Mexico. Science 331, 312–315 (2011).
Valentine, D. L. et al. Propane respiration jump-starts microbial response to a deep oil spill. Science 330, 208–211 (2010).
McNutt, M. K. et al. Review of flow rate estimates of the Deepwater Horizon oil spill. Proc. Natl Acad. Sci. USA 109, 20260–20267 (2012).
National Commission on the BP Deepwater Horizon Oil Spill and Offshore Drilling. The use of surface and subsea dispersants during the BP Deepwater Horizon oil spill. GPO [online], (2011).
Camilli, R. et al. Tracking hydrocarbon plume transport and biodegradation at Deepwater Horizon. Science 330, 201–204 (2010).
Diercks, A.-R. et al. Characterization of subsurface polycyclic aromatic hydrocarbons at the Deepwater Horizon site. Geophys. Res. Lett. 37, L20602 (2010).
Socolofsky, S. A., Adams, E. E. & Sherwood, C. R. Formation dynamics of subsurface hydrocarbon intrusions following the Deepwater Horizon blowout. Geophys. Res. Lett. 38, L09602 (2011).
Joye, S. B., MacDonald, I. R., Leifer, I. & Asper, V. Magnitude and oxidation potential of hydrocarbon gases released from the BP oil well blowout. Nature Geosci. 4, 160–164 (2011).
Reddy, C. M. et al. Composition and fate of gas and oil released to the water column during the Deepwater Horizon oil spill. Proc. Natl Acad. Sci. USA 109, 20229–20234 (2012).
Gray, J. L. et al. Presence of the Corexit component dioctyl sodium sulfosuccinate in Gulf of Mexico waters after the 2010 Deepwater Horizon oil spill. Chemosphere 95, 124–130 (2014).
Kujawinski, E. B. et al. Fate of dispersants associated with the Deepwater Horizon oil spill. Environ. Sci. Technol. 45, 1298–1306 (2011).
Atlas, R. M. & Hazen, T. C. Oil biodegradation and bioremediation: a tale of the two worst spills in U. S. history. Environ. Sci. Technol. 45, 6709–6715 (2011).
Dubinsky, E. A. et al. Succession of hydrocarbon-degrading bacteria in the aftermath of the Deepwater Horizon oil spill in the Gulf of Mexico. Environ. Sci. Technol. 47, 10860–10867 (2013).
Mason, O. U. et al. Metagenome, metatranscriptome and single-cell sequencing reveal microbial response to Deepwater Horizon oil spill. ISME J. 6, 1715–1727 (2012).
Redmond, M. C. & Valentine, D. L. Natural gas and temperature structured a microbial community response to the Deepwater Horizon oil spill. Proc. Natl Acad. Sci. USA 109, 20292–20297 (2012).
Valentine, D. L. et al. Dynamic autoinoculation and the microbial ecology of a deep water hydrocarbon irruption. Proc. Natl Acad. Sci. USA 109, 20286–20291 (2012).
Passow, U., Ziervogel, K., Asper, V. & Diercks, A. Marine snow formation in the aftermath of the Deepwater Horizon oil spill in the Gulf of Mexico. Environ. Res. Lett. 7, 035301 (2012).
Yin, F., John, G. F., Hayworth, J. S. & Clement, T. P. Long-term monitoring data to describe the fate of polycyclic aromatic hydrocarbons in Deepwater Horizon oil submerged off Alabama's beaches. Sci. Total Environ. 508, 46–56 (2015).
Lamendella, R. et al. Assessment of the Deepwater Horizon oil spill impact on Gulf coast microbial communities. Front. Microbiol. 5, 130 (2014).
Widdel, F., Knittel, K. & Galushko, A. in Handbook of Hydrocarbon and Lipid Microbiology Vol. 3 (eds Timmis, K. N. et al.) 1997–2021 (Springer Berlin Heidelberg, 2010).
Acknowledgements
The authors acknowledge the BP/Gulf of Mexico Research Initiative (GoMRI) for supporting the ECOGIG (Ecosystem Impacts of Oil and Gas Inputs to the Gulf; to S.B.J.) and C-IMAGE (Center for Integrated Modeling and Analysis of Gulf Ecosystems; to J.H.P) consortia. Additional support from the Guy Harvey Ocean Research Foundation (to J.H.P.) is also appreciated. This paper is ECOGIG contribution number 204.
Author information
Authors and Affiliations
Corresponding author
Ethics declarations
Competing interests
The authors declare no competing financial interests.
Related links
PowerPoint slides
Rights and permissions
About this article
Cite this article
Kleindienst, S., Paul, J. & Joye, S. Using dispersants after oil spills: impacts on the composition and activity of microbial communities. Nat Rev Microbiol 13, 388–396 (2015). https://doi.org/10.1038/nrmicro3452
Published:
Issue Date:
DOI: https://doi.org/10.1038/nrmicro3452
This article is cited by
-
C,N co-doped TiO2 hollow nanofibers coated stainless steel meshes for oil/water separation and visible light-driven degradation of pollutants
Scientific Reports (2023)
-
Impacts of dispersants on microbial communities and ecological systems
Applied Microbiology and Biotechnology (2023)
-
Microbial remediation of oil-contaminated shorelines: a review
Environmental Science and Pollution Research (2023)
-
Potential of some microbial isolates on diesel hydrocarbons removal, bio surfactant production and biofilm formation
Journal of Environmental Health Science and Engineering (2023)
-
Transport and behavior of marine oil spill containing polycyclic aromatic hydrocarbons in mesocosm experiments
Journal of Oceanology and Limnology (2023)